Rise of mammals and early hominins
Life appeared on Earth about 3.5 Gya. At first, bacteria dominated. In numbers they still do, but they are not alone. We will now take up where we left off after the K-T extinction and the beginning of the first epoch of the Cenozoic Era.
Paleocene Epoch, 65 Mya
Although the earliest true mammals evolved during the late Triassic, they remained small and relatively inconspicuous until around 65 Mya, the time of the K-T extinction and the disappearance of the dinosaurs. It Is not clear how mammals managed to survive. One theory, based on the hypothesis that dinosaurs were killed by extreme heat after a huge asteroid struck the Earth, would have it that mammals stayed safe either in their burrows or under the sea until the worst heat had passed. In this case, the heat would have had to pass quickly. Perhaps a more likely suggestion is that particles in the air, whether from asteroid impact or volcanic activity, reduced incident sunlight so that photosynthesis was diminished. Animals which depended on plants for food (or on animals dependent on plants) died out, whereas those which ate organisms like insects or worms, which in turn fed on detritus, survived. Possibly both processes – and others – contributed. Be that as it may, after the dinosaurs died out, surviving mammals could creep out of their holes to occupy the old econiches as well as new ones. The number of mammals and mammal species underwent an extraordinary increase.
Mammals are characterized by:
- having differentiated teeth, i.e., teeth differently shaped to fulfill different functions in different parts of the mouth (e.g., incisors, canines and molars);
- being endothermic, or warm-blooded, which allows them to adjust their body temperatures according to external conditions, an advantage for adaptation to different climates;
- bearing their young alive (in most cases);
- producing milk to feed their young (in mammary glands);
- having fur or hair on their bodies.
Three orders of mammals which survived are still around today. Monotremes are rather rare egg-laying mammals, such as the platypus. Remote ancestors of mammals all laid eggs and these still do. Marsupials, such as kangaroos and koalas, do not lay eggs, but their young are born underdeveloped and must be protected in the mother’s pouch as they grow and develop. Placental mammals, such as humans, protect their young within the mother’s body. They are the most divers and wide-ranging of contemporary mammals.
It is useful to show again the climate change graph from the last article.
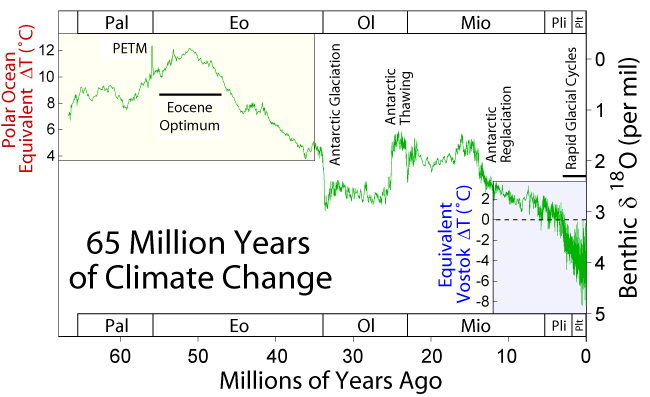
65 million years of climate change, from Wikimedia Commons
At the beginning of the Tertiary Period, climates were warm and forests spread across all the continents. Angiosperms, plants fertilized by insects, grew everywhere, so plants, insects and animals evolved together. Among animals, one group, the archonta, were the ancestors of today’s bats, flying squirrels, tree shrews and primates.
Eocene Epoch, 55 Mya
During the warmth of the early Eocene Epoch, plant life abounded. Placental mammals and the first primates appeared. As forests developed, mammals evolved to inhabit them, ranging over what are now Europe, North America, Asia and Africa. As greenery spread, photosynthesis increased and therefore so did the amount of oxygen in the atmosphere.
There are numerous candidate fossils for the first primates. They include Purgatorius (Montana, North America, c. 65 Mya, at the end of the Paleocene), Altiatlasius (Morocco, c. 55-56 Mya), Teilhardina (North America and Asia, c. 56 Mya), Notharctus (North America, c. 50 Mya) and Eosimias (China, c. 45 Mya). Altiatlasius is generally considered to be the oldest known primate, even though only a few molars and a piece of jaw have been found. Early primates were small, squirrel-like creatures, but their paws could grasp and their eyes looked forwards, thereby giving them improved stereoscopic vision, so they were well able to walk or run or climb in trees.
Oligocene Epoch, 33.9 Mya
As the Eocene warmth gave way to cooling, the Antarctic ice cap formed. Glaciations accelerated the cooling of temperatures and absorption of water, causing increased aridity. Forests regressed, leaving grasslands behind. The necessity of adaptation to new conditions brought about the disappearance of fauna in higher latitudes and the appearance of new ones. Mammals became larger, sometimes huge. It was at this time that the first modern monkeys evolved in Africa or in Asia, exactly where being a matter of some controversy. One such creature, the Aegyptopithecus, or Egyptian Monkey, lived in the forests of what is now Egypt around 35-33 Mya and possessed the gross anatomical traits of later monkeys. He weighed around 6 kg, lived mostly in trees and had opposable thumbs on all four feet, ideal for holding onto tree limbs.
In Western Europe, a sudden change in fauna known as the Grande Coupure involved the extinction of many species. The fossil trace of hominoids is then lost until the Miocene.
Miocene Epoch, 23 Mya
Around 23 Mya, an increase in temperatures was followed by a subsequent division of monkeys into two lines: One, the Cercopithecoidea, now taxonomically considered a super-family, gave rise to the Old World monkeys (catarrhines) remaining in Africa and Asia today. The other was Hominoidea, which gave rise to tail-less gibbons, orangutans, gorillas, chimpanzees and humans. Hominoids flourished, beginning what has been called the “Golden age of hominoids”. One early hominoid, Proconsul, 25-23 Mya, rather resembled a monkey but was definitely not one, being tailless and having a larger brain and ape-like teeth. Later, 17 Mya, Morotopithecus could move in a vertical stance, suspended from tree branches (brachiation). This technique may later have been transferred to the ground to give upright walking.
With the closing of the Tethys Sea around 16 Mya, African simians could migrate to Europe and Asia. Except for orangutans in Asia, all but those remaining in Africa eventually became extinct.
Several hominoid fossils have been found dating from around 14 Mya. One, Kenyapithecus sometimes called Afropithecus), had thickly enameled teeth and may have been an ancestor of modern hominoids.
Subsequent, faster glacial fluctuations reduced the size of northern forests and brought about a rapid diminution of the relative numbers of tailless hominoids compared to cercopithecoids. In fact, hominoids barely survived; 8 Mya, only a few species remained. In Asia, only the ancestors of todays orangutans and gibbons made it. The only other hominoid survivors were in Africa and are dealt with in the next section. Today there remain only five hominoid species for 80 species of Old World Monkeys. Even in Africa, they were greatly outnumbered by cercopithecoids.
After Kenyapithecus, few fossil hominoids occur before the arrival of the australopiths, leaving a 7‑My fossil-less period known as the “African ape gap”. Nevertheless, more fossils are turning up all the time. Samburapithecus, from 9.5 Mya, probably lived before the LCA,[ref]The last common ancestor of chimps and humans, if you have forgotten.[/ref] which the molecular-clock method dates to between 6 an 8 Mya.
Possible and probable hominins
Detailed descriptions of the various hominin species and their characteristics do not make for an exciting literary experience for many people, but this is unavoidable. There is much lack of certainty in the details of this domain, but a general evolutionary trend is perfectly clear.
As the above figure shows, the climate since c. 10 Mya has been one of cooling and therefore drying. Some forests have given way to grasslands, like present-day African savannas. At the time when today’s hominin fossils were alive and thriving, the area was what paleontologists call a “mosaic” – a mixed regions of woods, savannas and riverside forests. So creatures living there would have benefited from living both in the trees and on the ground. That is what they did.
Those readers wishing to ignore the details can skip from here to the paragraph “Homo sapiens”.
To date, four species have been discovered of which one may be the first hominin. All are somewhat controversial.
The oldest, Sahelanthropus tchadensis (also referred to as “Toumai”), dated about 7-6 Mya[ref]Some authors cite slightly different dates. In general, we use those of the Smithsonian Human Origins Program, http://humanorigins.si.edu/research.[/ref], lived in what is now Chad, which was then a mosaic environment and not yet a desert.[ref]It goes without saying that the statement that a species “lived” in a place at some time means that its fossils have been found at that place and dated to that time.[/ref] Only a cranium and several mandibles have been found. The cranium is no bigger than a present-day chimp’s, with a small brain and large brow ridges, but its sloping face, its small canines and enlarged cheek teeth arranged in the shape of a C, and the position of the foramen magnum (the relative importance of which is disputed in this case) suggest that it was a hominin, not an ape, and capable of walking upright or living in trees. In this case, it came (probably just) after the LCA, so the fact of its having lived west of the Great African Rift negates the thesis (“East Side Story”) that hominins evolved in eastern savannas where their upright posture, enabling them to see over the grass, could have been an adaptive trait. All hominoids, chimps and all, are capable of walking upright, but can not do so as fast or as far as humans.
Of Orrorin tugenensis, who lived in Kenya about 6.2-5.8 Mya, only some teeth, a femur and some phalanges have been found. These fragments indicate that he could live well in trees but was capable of frequently walking upright. His teeth were thickly enameled like those of later australopiths, but smaller. The thick enamel may not be unique to hominin-like creatures and some paleontologists question even whether Orrorin was a hominin.
Two forms of Ardipithecus have been found. One, Ardipithecus kaddaba dates from 5.8-5.2 Mya and rather resembles a chimpanzee, but was capable of walking upright.
The more recent one, Ardipithecus ramidus, (“Ardi”) dates from around 4.5 Mya in what is now Ethiopia. Although his foramen magnum was somewhat farther forward than that of chimps, he still was more like a chimpanzee than a hominin, although he had a U-shaped jaw, unlike apes. A reconstruction of a crushed pelvis indicates Ardi was at home in trees or on the ground, probably more the former, as his hands were those of a tree dweller. Nor could he run long distances upright. He may represent an additional line of hominoids to pangins and hominins, or he might lie along the line to Australopithecines. Whether or not he was a direct ancestor of man is debated. We will shortly have more to say about Ardi.
Archaic and transitional Homo
This rather large group includes the sub-tribe Australopithecina and its two genera, the earlier Australopithecus and the later Paranthropecus. Although the skulls and overall size of australopiths were chimp-like, their front teeth were relatively smaller and their dentitions starting to approach the parabolic shape of those of humans. Also, australopiths could walk upright. They therefore show both ape-like and hominin characteristics, putting them on the road from the former to the latter.
Paranthrops had huge teeth and massive jaws.
Australopithecus hominins
Australopithecus lived during a warm climatic period in a mosaic environment of forest and savanna in east and south Africa between about 4.2 and 2.5 Mya, a period of well over a million years. Members of this genus generally had well developed mandibles and teeth for eating tough roots and plants and they were used to walking upright as well as moving about in trees. Their brains were not significantly bigger than chimps’ brains, although they may have been organized differently. There are at least five species considered to be members of the genus Australopithecus; they are a rather heterogeneous lot.
Australopithecus anamensis lived in current-day Kenya about 3.85-2.95 Mya. He could walk upright or move about in trees. His teeth resembled more those of humans than of chimps. Opinions about him differ:
- Some paleontologists think Au. anamensis may be the ancestor of Au. garhi who in turn would be ancestor of Homo.
- Fossils bones of Au. anamensis also have been found in Ethiopia, where he lived at the same time as Ardipithecus ramidus. This suggests that he may have been a descendant of Ardi and ancestor of Au. afarensis, as is also suggested by his parabolic jaw structure.
- Others wonder if he is not simply the same species as Au. Afarensis.
The diverging opinions aboutf Au. anamensis are a good example of the current state of uncertainty concerning hominid fossils.
Australopithecus afarensis lived in Ethiopia and Kenya roughly 3.7-3.0 Mya and so lived over a period of about a million years. Many bones of this species have been found, including the so-called “First Family”. With an ape-like face and cranium and long arms for climbing in trees, but small canines and definite bipedal capabilities in the knee and hip bones, she possessed a mixture of ape-like and hominin characteristics which enabled her to survive important environmental changes. Fossil remains of over 300 individuals have been found. Not only was she one of the longest-lived hominids, she is also the best known, because of Lucy. If there is a common “spokesperson” for australopiths in general and afarensis in particular, it is certainly Lucy, the 40% complete skeleton of a young afarensis woman found in Ethiopia. Her fame as a hominin fossil has made the tour of the world.
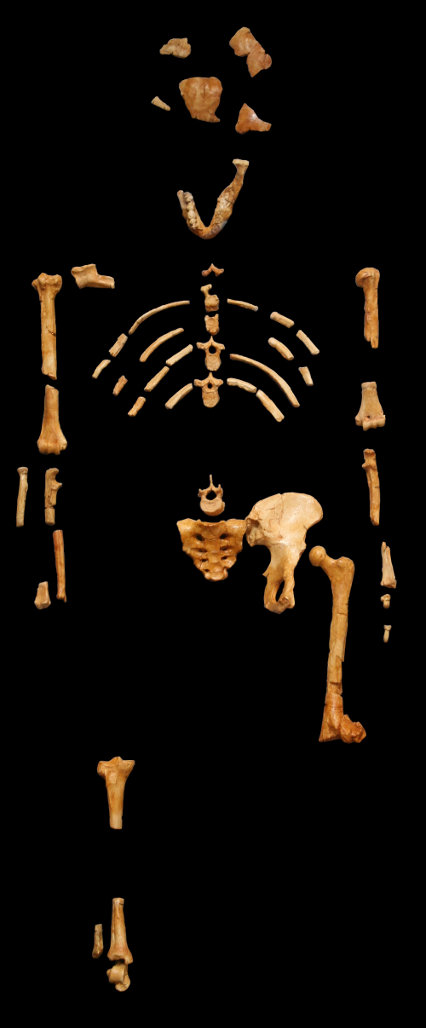
Lucy’s skeleton. Cast from Museum national d’histoire naturel, Paris. Photo from Wikipdedia Commons
Although Lucy could and did walk upright, she certainly had an awkward, swaying gait. Her brain was somewhat larger than a chimp’s and she probably used natural objects which she found, like sticks and stones, for tools.
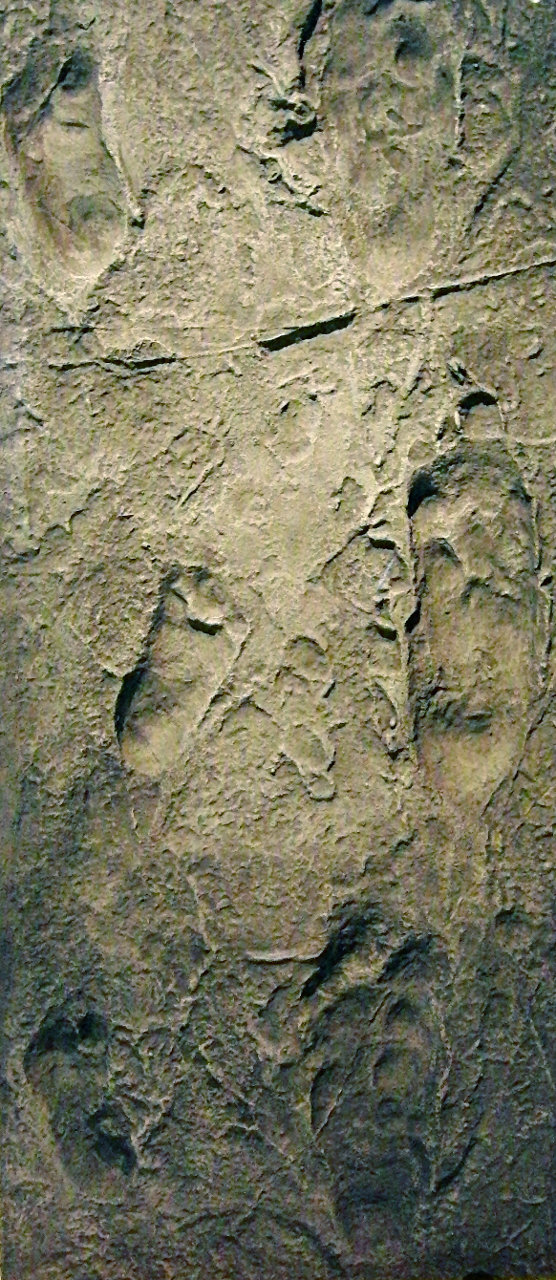
Partial copy of Laetoli footprints. Replica in National Museum of Nature and Science, Tokyo. Photo from Wikipedia Commons
Footprints of two hominins from the same period, about 3.6 Mya, have been found at Laetoli, in Kenya. The big toe of the walkers is in line with the foot and the walk is a heel-first-toes-last walk which suggests either modern humans or Australopithecus to different studies. Since the observed short stride corresponds to the short legs of Au. afarensis, some of whose bones have been found nearby in the same sediment layer, it is generally accepted that the prints are of that species. Even so, some scientists insist the footprints are those of Au. anamensis.
On the basis of these footprints, it has been suggested that bipedalism did not evolve from quadripedalism, but had always been possible and was instead lost by monkeys and others who became uniquely quadripedal.[ref]Yvette Levoisin, “L’homme de descend pas d’un primate arboricole!”. http://www.hominides.com/html/references/bipede-homme-primate-deloison.php.[/ref] The least one can say is that this idea does not seem to have caught on very much.
Another skull from about 3.5 Mya, which has been named Kenyanthropus platyops, is controversial. One paleontologist thinks it is just a deformed skull of Au. afarensis; another, an ancestor of Homo rudolfensis. However, a recent discovery of what would be the oldest known stone tool dates back to 3.3 Mya.[ref]“Wrong Turn Leads to Discovery of Oldest Stone Tools”, http://news.nationalgeographic.com/2015/05/150520-oldest-stone-tools-discovery-harmand-archaeology/[/ref] It was found near the site of Kenyanthropus, so it may have been used by him, whatever he was.
Australopithecus africanus is similar to Au. afarensis in possessing both ape-like and human-like features, so much so that some paleontologists consider them to be the same species. It lived in east and south Africa 3.3-2.1 Mya. It had teeth more like those of humans than of australopiths, with smaller canines arranged in a semicircle. It had a flat face and the foramen magnum placed as for an upright posture. Its brain was bigger than that of Au. afarensis and it was both bipedal and arboreal. So it is considered by some to be an ancestor of Homo.
Australopithecus bahrelghazali (called “Abel” by his discoverers) lived about 3.5-3 Mya in Chad, west of the Rift. Many paleontologists think he is just a geographical variant of Au. afarensis. Its discoverer, of course, does not agree.
Of Australopithecus garhi, who lived about 2.5 Mya in Ethiopia, only pieces of a skull have been found. Although a nearby partial skeleton may go with the skull, this is not yet proven. Although it might just be an Au. afarensis or a female Paranthropus aethiopicus, it was considered to represent a separate species because of the previously unknown combination of a small brain and large molars. Bones found nearby indicate that a sharp-edged tool had been used to remove meat.
The most recent australopith, both for its life period and its discovery, is Australopithecus sediba, who lived in south Africa between 1.977 and 1.98 Mya.[ref]”Australopithecus sediba – new analyses and surprise”, Smithsonian Human Origins Program, http://humanorigins.si.edu/evidence/human-fossils/species/australopithecus-sediba[/ref] Au. sediba has certain details of its teeth, arm and leg length and upper chest like earlier australopiths, but other tooth traits and lower chest like humans. Its discoverers think it is descended from Au. africanus, and that it shows features more like Homo than like any other australopith. For them, it could help understand the transition from late australopiths to direct ancestors of humans, in which case it could link the origin of humans to South rather than East Africa. As usual, not everyone agrees. A study of its teeth finds it to be distinct from east African australopiths but close to south African Au. africanus. On the other hand, a study of its jaw finds it to be distinct from Au. africanus. Although it was bipedal, it had what is described as a hyper-pronating gait, meaning that its feet rolled inward at the end of each step.
So there are two lines suggested — and debated:
- one in east Africa (Ardipithecus ramidus → Au. anamensis → Au. → afarensis → Homo);
- one in south Africa (Au. africanus → Au. sediba → Homo).
Paranthropus hominids
Though this group was originally thought to be australopiths, they are now considered to be a separate species because of their more robust frame, in particular, their chewing apparatus – jaw bone and muscle, and teeth. They are a more homogeneous group than australopiths.
Paranthropus aethiopicus lived 2.7-2.3 Mya in East Africa. A jawbone and a skull have been found, the latter with a protruding face, strong jaw and well developed sagittal crest. But its most striking feature is a set of huge, thickly-enameled megadont teeth in a powerful jaw attached via large zygomatic arches to a sagittal crest in order to permit the chewing of tough, fibrous foods. This guy could eat really tough things like roots. Some paleontologists think he was a robust form of Australopithecus, maybe intermediate between Au. afaransis and P. robustus.
Paranthropus boisei (originally called Zinjanthropus boisei, “Zinj” for short) lived 2.3-1.2 Mya in East Africa. His skull has a massive jaw, megadont teeth – even bigger than those of P. robustus – and flaring cheekbones to hold his strong chewing muscles. He is often referred to as “Nutcracker man” and one study indeed finds that he ate nuts. His brain was bigger than that of his predecessors and increased gradually in size over time, as he flourished for about 1 million years. One hypothesis is that he evolved from P. aethiopicus. He is generally considered to be a side branch of our evolutionary tree because he lived in east Africa at the same time as the first Homo species.
Paranthropus robustus, lived 1.8-1.5 Mya in South Africa. He had an imposing, wide face, with large zygomatic arches, a sagittal crest and robust, almost megadont jaws for chewing tough fibrous foods. He may have been the user of bone tools found nearby.
General appearance of Australopithecus and Paranthropecus
If you met an Australopith in the street, you would wonder why he was loose. Even if he were wearing a suit, you would probably call the nearest zoo or circus to inform them that one of their stars had escaped. They rarely reached 1.4m in height. Their brains were small and they were certainly covered in fur and had chimp-like faces with protruding muzzles. Even if the one you saw walked on two legs, you would have thought he was walking on his hind legs, because his “arms” more resembled front legs, with his hands pretty much like his feet. And his way of walking would probably make you wonder how long he had been down out of his tree – or whether you were out of yours.
Paranthropus specimens were slightly taller than early Australopiths. Still, if you met one in the street you would take one look at his massive jaw and choose a different street.