Muscles — motility and energy
The most obvious distinction between plants and animals is animals’ motility, our ability to move around whenever we feel like it.
Scientists think that the basic mechanism of motility is similar in all complex cells. This mechanism and its evolutionary origins were referred to briefly in the section on the cytoskeleton in the biochemistry chapter.
Motility of large organisms requires muscles. There are three types of muscles:
- Skeletal muscles are attached to bones by tendons and contract to produce movement of the body. They are innervated voluntarily. (This is partly a question of definition. Even when they are innervated involuntarily, the innervation is said to be voluntary.
- Cardiac muscles make the heart beat. In fact, the heart is pretty much a big muscle. The heart has its own clock, whose rate can be modified by the nervous system.
- Smooth muscles line the digestive and respiratory tracts, surround sphincters and control the opening of the iris of the eye, among other things. Their action is involuntary, triggered by hormones, the ANS and local conditions.
Let’s look at those in more detail.
Skeletal muscles
Skeletal muscles do not just make joints move. Muscles are rarely completely relaxed, but maintain muscle tone, constantly contracting and relaxing by small amounts in order to stabilize joints and maintain balance. This is brought about by complex interactions with the nervous system.
During the development of skeletal muscles, many individual cells fuse together, usually retaining their nuclei and mitochondria. Multiple mitochondria are necessary in order to supply the great amounts of energy needed by muscles. Muscles which work more and contain more mitochondria are darker colored than those which contain fewer. Chicken legs serve to walk and are dark meat; chicken wings serve little purpose and are small and light-colored.
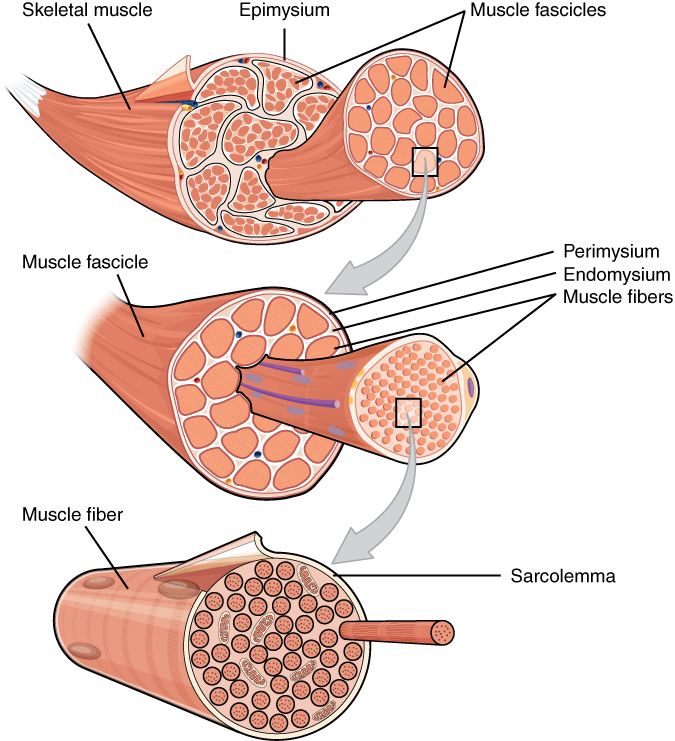
Three skeletal muscle structures, from Openstax College
Looking from the outside in, muscle cells consist of numerous components, one nested within another, including three layers of connective tissue, or mysia (singular mysium).
- An outer layer, the epimysium, holds the muscle together as it contracts and expands. It also connects to tendons.
- Inside the epimysium are bundles of cells called fascicles, bounded by a second layer of connective tissue, the perimysium.
- Inside each fascicle, the muscle cells themselves, called fibers, are bundled in another layer of connective tissue, the endomysium. The endomysium furnishes nutrition to the cells. Fibers can be quite long, up to 30 cm in the leg.
- The fibers in turn are bundles of elements, the myofibrils, which run the length of the fiber.
Within the fibers, skeletal muscle cell components have special names, beginning with “sarco” (Greek for “flesh”). The cell membrane is called the sarcolemma; the cytoplasm, the sarcoplasm; and the smooth endoplasmic reticulum, the sarcoplasmic reticulum (abbreviated SR)
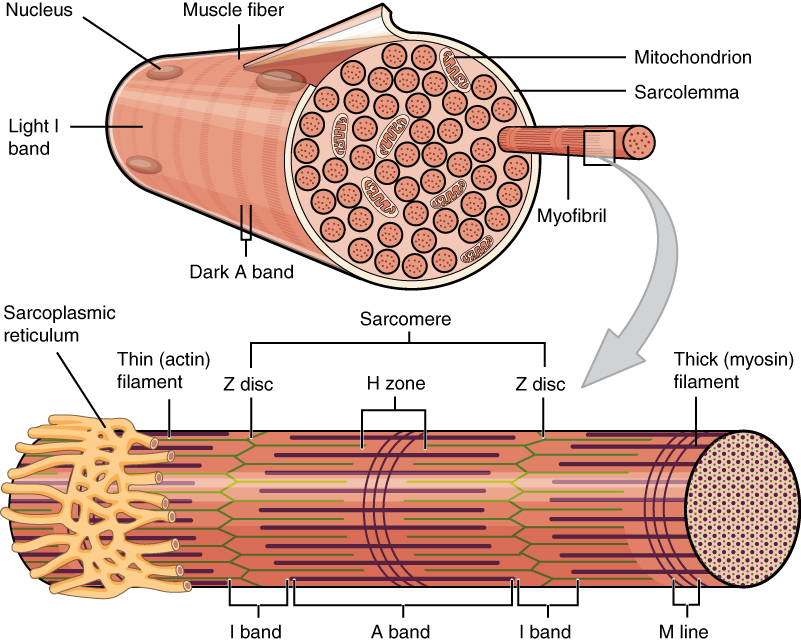
Muscle fibers, from Openstax College
Skeletal muscles appear to be striated (or striped) because each myofibril is composed of a series of elements called sarcomeres, which are where the action is. The sarcomeres are placed end to end along the myofibril and contain two types of filaments: thin filaments called actin and thicker ones called myosin. The actin filaments are anchored to the ends of the sarcomeres (called Z disks or Z lines) and do not meet in the middle. The myosin filaments are positioned at the mid-point of the sarcomere, called the M line, and each end is bound to the nearest Z disc by elastic bands of the protein titin, or connectin, which act like springs, allowing the sarcomere to expand and contract while maintaining its form. The actin and myosin filaments overlap partially.
The myosin filaments are composed of multiple myosin molecules wound up together. Each molecule has a head on one end and this protrudes outward from the axis of the filament. Each head has a binding site for actin on the end and another one for ATP. In fact, the myosin head functions as an ATPase enzyme and can cleave ATP to recover the energy in it. The energy is then available for powering muscular contraction.
The actin filaments, consisting similarly of wound-up cables of actin molecules, contain binding sites for the myosin heads. When the muscle is relaxed, these sites are blocked by the protein tropomyosin, which spirals around the actin and is in turn is bound with another protein, troponin.
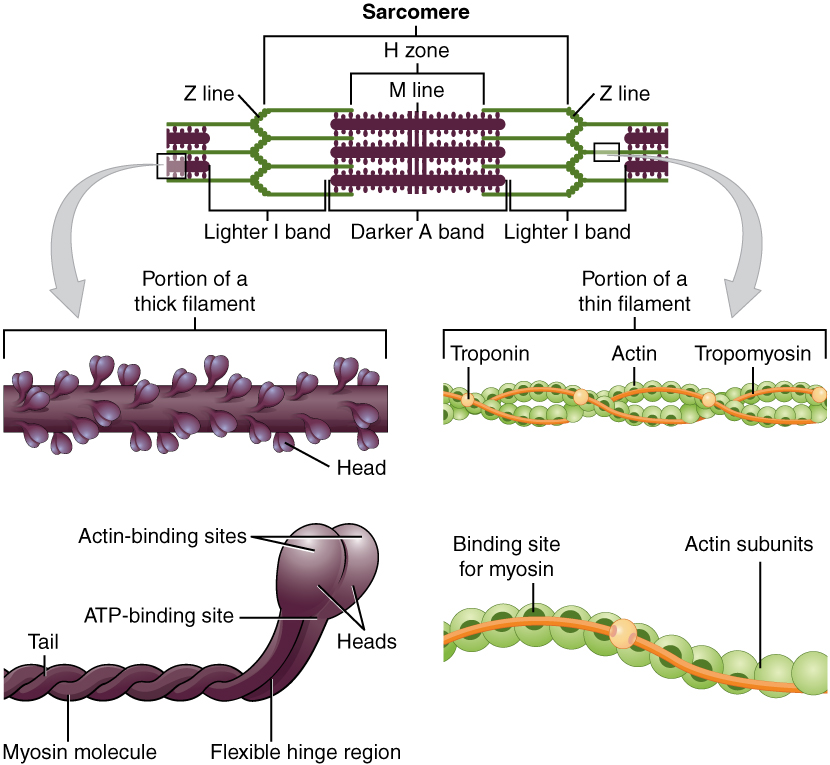
Thick and thin muscle filaments, from Openstax College
The neuromuscular junction (NMJ) is the point at which the motor neuron from the peripheral nervous system innervates the fiber Each fiber is connected to one branch of a motor neuron axon[ref]An axon is the output arm of a neuron. This will be discussed in some detail in the neuroscience chapter.[/ref]. If more than one fiber is connected to a neuron, the set of fibers for that neuron constitutes a motor unit. This allows for precise innervation of muscle fibers: the smaller the motor unit, the more precise the control. In the thousands of muscle fibers which move our eyeballs, a motor unit is made up of only about six fibers, making for great precision of movement. In contrast, in our back or thigh muscles, a motor unit may include thousands of muscle fibers.
When the NMJ receives an action potential, it releases the neurotransmitter acetylcholine, abbreviated Ach. Reception of Ach on the fiber receptors brings about the propagation of an action potential along the sarcolemma and through so-called T tubules to the SR, which opens calcium channels and releases Ca++ into the cell.
In more detail, the Ach receptors contain Ach-gated cation channels which open when Ach binds to them. Since the cell is normally at a negative voltage relative to its surroundings, opening the channel lets positive ions flow through the membrane and into the cell, causing it to depolarize (become less negative). This causes the opening of voltage-gated sodium channels, so Na+ enters the cell and brings about an action potential. (For more information on action potentials, see the biochemistry chapter.) When the action potential crosses the T tubules and reaches the SR, the SR releases Ca++ ions into the cell. The Ca++ binds to the troponin, which in turn causes tropomyosin to re-configure in such a way that the myosin binding sites on the actin are unblocked.
What happens next is described by the sliding-filament model of muscular contraction. (Some details of this model are still hypothetical.)
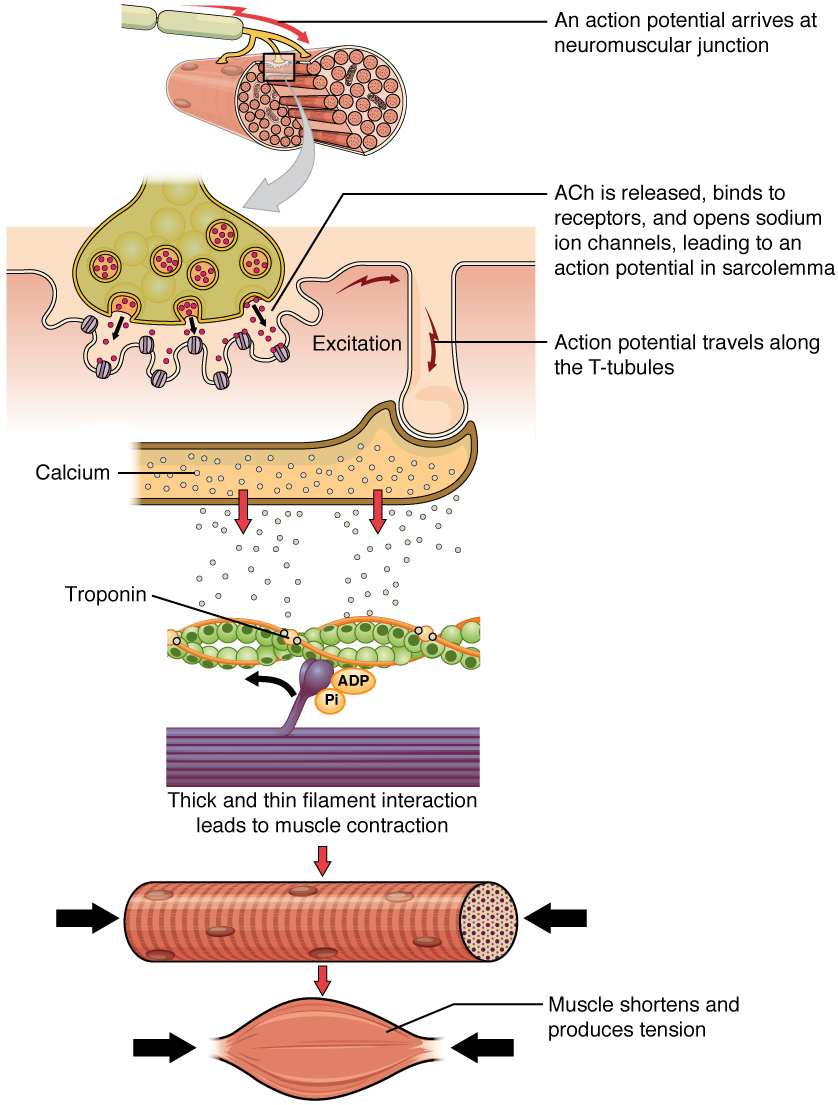
Muscle fiber contraction, from Openstax College
- The myosin heads already are bound to ADP and Pi. As the Pi is released, the myosin heads bind strongly to the actin, creating cross-bridges between the two filaments.
- The myosin heads then execute the power stroke: They bend toward the M-line (therefore in opposite directions on each side of the M-line), dragging the actin filaments with them (about 10 nm), increasing the overlap of the two filaments and compacting the sarcomere and, hence, the muscle.
- The myosin head now can bend no more. ATP binds to it and causes it to detach from the actin. The head, using energy from conversion of the ATP to ADP and Pi, returns to its neutral position. It now is “cocked” and ready to launch another power stroke, As long as the binding sites on the actin are unblocked the cycle can repeat.
Eventually, the original Ca++ is stored back into the SR by a membrane pump. Then the cycle stops until more Ca++ unblocks the actin binding sites.
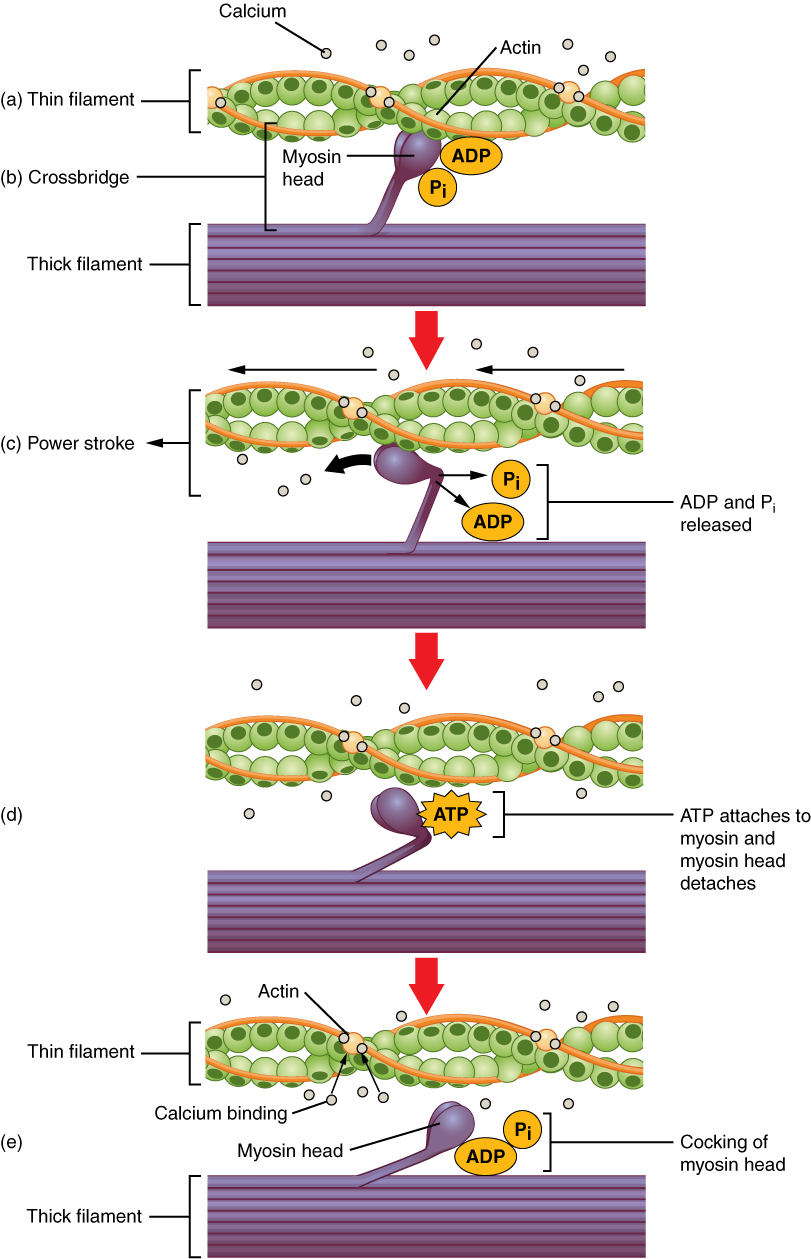
Sliding-filament steps, from Openstax College
In the sarcomere, the myosin heads drag the actin filaments along, step by step, as they overlap and the sarcomere shortens.
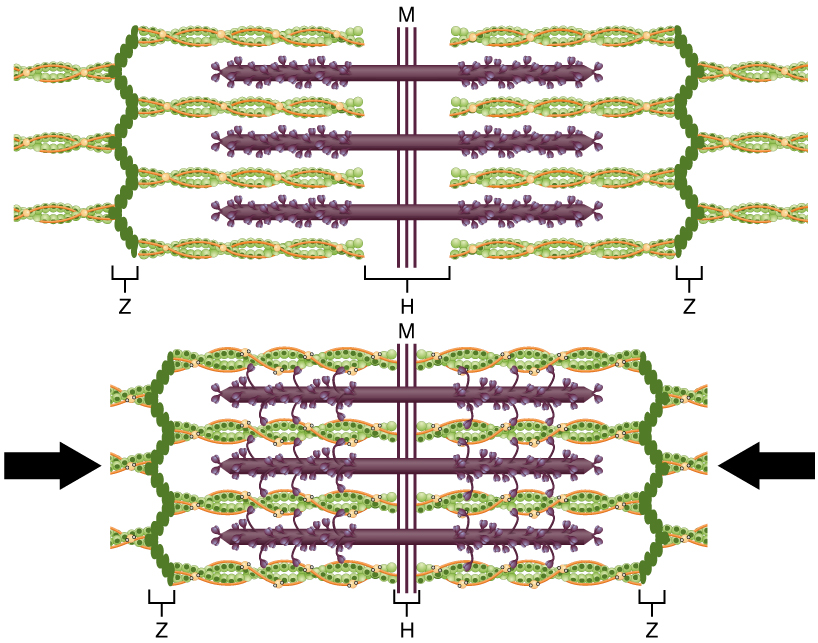
Sliding filaments, from Openstax College
Energy for muscle action
Muscles need energy in order to function. This is why skeletal muscles have multiple mitochondria, energy factories. Energy can come from different sources and the muscles which use different sources differ in their type and function.
When a muscle is at rest, excess ATP produced may be transferred for storage to creatine phosphate (or phosphocreatine) in the muscle. This molecule stores energy in its phosphate bonds and can give it back very quickly, but can not store more than about 15 seconds worth of energy. So it is a fast, but short-duration source of energy.
Energy can also be obtained without the need of oxygen by anaerobic respiration through the conversion of glucose into ATP by the process of glycolysis, which we have already considered in some detail. This procedure is slower and, as we have seen, relatively inefficient.
After this, if oxygen still is not available, fermentation, already discussed, can convert pyruvate into lactic acid with a small gain in energy.
If oxygen is present, then aerobic respiration (Krebs cycle + oxidative phosphorylation), which is very efficient, provides energy. But it does require a regular supply of oxygen and is slower than glycolysis. So intense energy needs by muscles will first be supplied by phosphocreatine and then by glycolysis.
When muscles need ATP from aerobic respiration but the oxygen supply is short, then there is what is called an oxygen debt, which makes us breathe hard after an intense muscular effort.
Cardiac muscles
Heart muscles are striated like skeletal muscles, but are shorter and only have one nucleus per cell, although they still have many mitochondria. They are multi-branched and the branches are interconnected through intercalated discs. Gap junctions between fibers allow rapid transmission of action potentials for coordination of activity throughout the heart. The heart has its own clock and is not controlled by the nervous system.
Muscle contraction occurs through a sliding- filament scheme similar to the one in skeletal muscles. But cardiac muscles are autorhythmic: Their contractions are controlled by pacemaker cells within the heart itself, although the ANS[ref]The autonomic nervous system, remember?[/ref] may signal the heart to speed up or slow down. The pacemaker cells cause a group of cells, called a functional syncytium, to auto-excite and create an action potential. This subject will be covered in the next chapter.
Smooth muscles
Smooth muscles are not striated and contain no sarcomeres. They occur in many organs and body structures, including the digestive, urinary, respiratory, circulatory and reproductive systems, so they are quite wide-spread. They are fusiform in shape – thick in the middle and tapered at the ends. Their action is involuntary, triggered by hormones, the ANS and local conditions.
They too contract through a system similar to the sliding-filament scheme of skeletal muscles, but with important differences. Smooth muscles possess no troponin-tropomyosin complex. In a process beginning with Ca++ ions binding to a protein called calmodulin, the myosin heads are activated by being phosphorylated. The heads crawl along the actin thin filament, which is attached at its ends to dense bodies, the smooth-muscle equivalent of Z discs. But this pulls on a network of intermediate filaments, which in turn contracts the whole fiber along its length, causing it to get shorter as it swells up in the middle.
So muscles can contract because of energy from oxidative respiratioin (mostly) which uses input from food which comes from … plants — by photosynthesis.