Hearing
Hearing depends on ionotropic mechanoreceptors based on selectively depolarizing ion channels. First, sound energy is reflected and directed by the earlobe (pinna) , which can detect some vertical direction and modulates the frequency some as a function thereof. Then the energy passes through the auditory canal of the so-called outer ear, whose sensitivity is subject to resonance at the mid-tones we use to distinguish sounds. At the end of the canal, the sound wave hits the eardrum (tympanic membrane). This membrane is connected to the trio of tiny bones – the hammer (malleus), anvil (incus) and stirrup (stapes) – which use physical leverage to bring about slighter but stronger movement where the stirrup presses against the entry membrane to the cochlea, the oval window. The cochlea is rather like a nautilus shell, larger at the entry and spiraling somewhat to a smaller end. Its walls are lined with fine hairs which have finer hairs upon them, called cilia. As the cilia are moved by the sound energy, they stretch the hair-cell membrane, causing the stretch-sensitive mechanoreceptors in the membranes to depolarize. The resulting action potential sends information about the frequency and amplitude of the sound along the auditory nerve. The wider entrance of the cochlea detects higher frequencies, the small end, lower, so that frequency is a function of position in the cochlea.
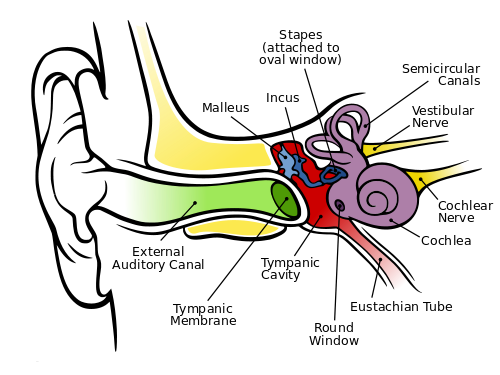
The human ear, by Chitta L. Brockmann, via Wikimedia Commons
Also visible in the figure are the three semicircular canals which constitute the vestibular system. They are oriented so that they can detect rotation and angular acceleration of the head about three axes and, so, in three dimensions. Not shown are the utricle and saccule, otolith organs which detect not only head tilts but the force of gravity. They and the semicircular canals are also lined with cilia and the set of vestibular and auditory cilia on either side of the head is said to constitute a vestibular labyrinth. Nerves from these systems are used by different parts of the brain in conjunction with ocular information and proprioception to detect and maintain the body’s orientation. They also contribute to the vestibulo-ocular reflex (VOR), which enables us to keep our eyes on a subject in spite of head movements.
The signals detected by the cilia are sent through other structures and finally arrive in the inferior colliculus of the thalamus on the opposite side of the brain. The thalamus relays the information on to the primary audio cortex, A1, in the superior temporal lobe, on whose surface location is a function of frequency, rather like the skin (somatosensory) map. It is not completely understood what happens after that. It is known though that two areas on the left side of the brain are important.
- The zone called Wernicke’s area is necessary to the understanding of language.
- Similarly, Broca’s area is necessary for producing speech.
Although these two areas are found on the left side of the brain in most right-handers, the right side also seems to be necessary for the comprehension of prosody (change of tonality and rhythm in speech). So it is only logical that the right side appears to play a role in the appreciation of music.
Detection of sound direction, though less good than in the case of vision, is accomplished vertically by the pinna, as already discussed, and horizontally by the difference in arrival time and intensity of the sound in the two ears.
Touch
Touch, or somatosensory perception, takes place in ionotropic receptors mostly in the dermis, the lower part of the skin. The outer part, or epidermis, is composed of sloughed-off dead cells from the dermis and constitutes a mechanical protection layer. The receptor cells themselves are in the dorsal root ganglion, a concentration of cells just posterior to the spinal cord. Such a cell has no dendrites and only one axon, which bifurcates, one end going to the dermis where its end holds the receptor, one into the spinal column. From there, it connects to interneurons which carry the signal up the spinal column to the thalamus on the opposite side of the brain from the receptors. In addition, the dermis contains free nerve endings with ion-channel receptors sensitive to temperature, extreme force or other possibly dangerous contacts.
The ventral posterior nucleus of the thalamus relays the signal to the somatosensory cortex (parietal lobe) just behind the central sulcus, where it is localized on a skin map, as already seen. In the map, the signals from a fairly well-defined part of the skin (such as the face or a hand) are found together. Outputs from the map connect to memories of previous touch-associated events. Cross-talk between adjacent areas of the map may lead to confusion as to the source of a stimulus.
Smell (olfaction)
The olfactory bulb in the top of the nose canal contains about 10 million olfactory receptors of about 1,000 types. They are metabotropic receptors on the cilia of the bulb. A smell is detected by many receptors and is distinguished by something like a weighted sum of the different results.
Olfaction is the only one of our senses whose receptors do not send information to the brain by way of the thalamus. Instead, they project to several sites in the cortex, including the olfactory cortex, which has direct influence on various brain areas. Information also passes via the olfactory tubercle to the thalamus, which transmits it to the orbitofrontal cortex in order to bring about consciousness of the odor. Other parts of the cortex control unconscious or reactive odor detection and memorization of smells.
The reason the olfactory receptors bypass the thalamus is evolutionary and is probably due to the fact that our ancestors already had a highly developed sense of smell, much like many animals today. So all that was left was to relay the smell to the thalamus in order to it to become conscious.
The receptors also send information to, among others, the amygdala for emotional response (e.g., for spoiled food) and to the hippocampus for memorizing of smells.
Taste
The surface of the tongue has structures called papillae, in which there are four types of receptors, only three of which are taste buds, containing about 10,000 taste cells. Each cell has several receptors responding to five different basic tastes: sweet, salt, bitter, sour (acid) and umami (meaty). Some are ionotropic, some metabotropic.
Signals pass from the receptors through another structure, the nucleus of the solitary tract (NST), to the thalamus either as pure basic tastes or mixtures. The thalamus relays it to the cortex for consciousness of the taste. Connections with the amygdala allow recognition of tastes associated with bad experiences in the past and so to be avoided. The NST, which influences the ANS, also receives input which may signal that you have eaten enough of that taste.
We distinguish different tastes far less well than different smells. Taste information is joined with olfactory information in the orbitofrontal cortex to generate flavor. Flavor therefore suffers if, for instance, our olfactory mucus is affected by a head cold.
Now, onward to brain output to the motor system.