We will consider the circulatory system only from a relatively high-level (undetailed) view of its structure and function – as a kind of plumbing system. This consists of several parts – the pump (the heart), the pipes (the veins and arteries) and the fluid (the blood).
The heart as pump – circulation
The heart consists of four chambers, two smaller, upper chambers – the atria (singular atrium) – and two larger, lower ones – the ventricles.
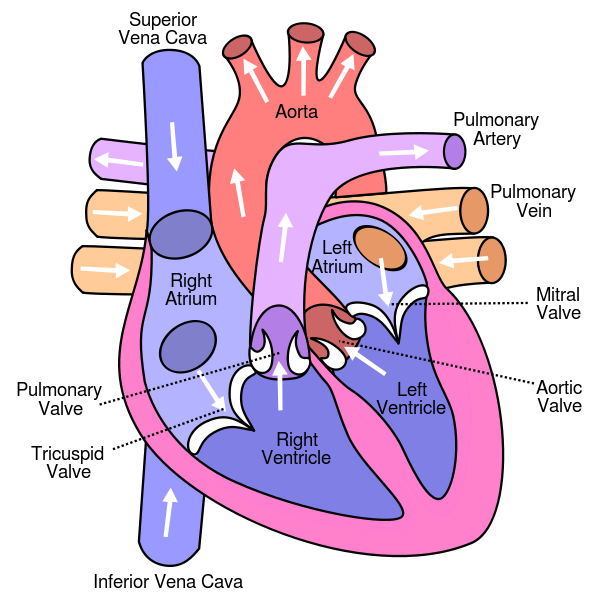
Structure of the human heart, from Wikimedia Commons
Even though we already indicated in the chapter on bioenergetics the general flow of blood through the heart and the cardiovascular circulatory system. it is worth repeating.
Oxygen-depleted blood from the body enters the right atrium of the heart and is pumped into the right ventricle and thence through the pulmonary artery into the lungs. (The figure is somewhat confusing because the lungs are shown above the heart, about at the place of the brain.) After picking up oxygen in the arterioles of the lungs, the blood returns through the pulmonary vein into the left atrium of the heart. It then is pumped into the left ventricle and out into the body. In capillaries, oxygen passes into cells and the oxygen-depleted blood is then returned to the right atrium through veins and either the superior or inferior vena cava.1Remember, veins flow towards the heart, whether they contain oxygenated blood or not. Four valves – the mitral, tricuspid, pulmonary and aortic – keep the blood from flowing back in the wrong direction.
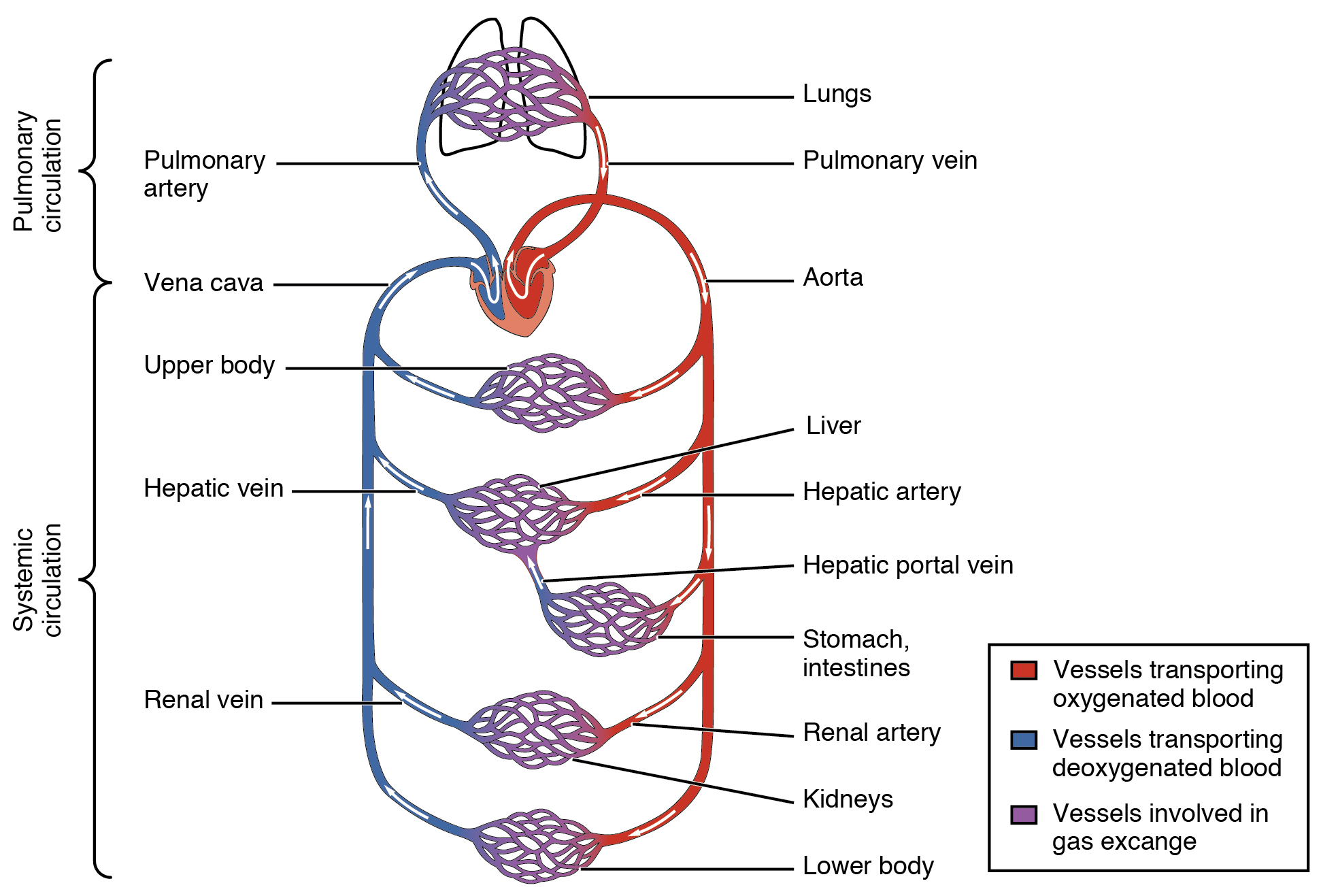
Cardiovascular circulation, from Openstax College
The walls of the heart are composed of three layers of tissue, of which the most important is the myocardium. This is the muscle which contracts to squeeze the four chambers and force blood through the heart and out into the lungs or body. Being mostly muscle, the heart itself requires energy and nutrients and, so, blood. This is supplied by the coronary arteries branching off the aorta, the main exit for oxygenated blood. The heart pumps blood to itself.
Electricity in the heart
Where study of the heart becomes really interesting is its electrical operation, where we meet our old friends energy and communications again.
The big question is, “What makes the heart beat?” Surprise: The commands to make it do so do not come from the brain. The brain can only modulate the rate of heartbeat.
The generation of an electrical signal to make the heart beat, i.e., to cause its muscles to contract in a rhythmic and well-synchronized manner, takes place in the sinoatrial (SA) node (or SAN, or sinus node) in the wall of the right atrium near the entrance of the superior vena cava. (See following figure.) Although similar signals may be generated elsewhere in the heart, they are usually overwhelmed by the higher-frequency SA signal. Like most cells in the body, the cell membranes of heart muscle contain a number of structures for allowing – or requiring – substances to enter or leave the cell. (We considered this in the biochemistry chapter.) Among these are Na/K pumps which maintain a negative voltage inside cell walls. In the SA node, however, instead of a voltage of around -80 Mv, slow leakage limits the voltage to -55-60 mV.
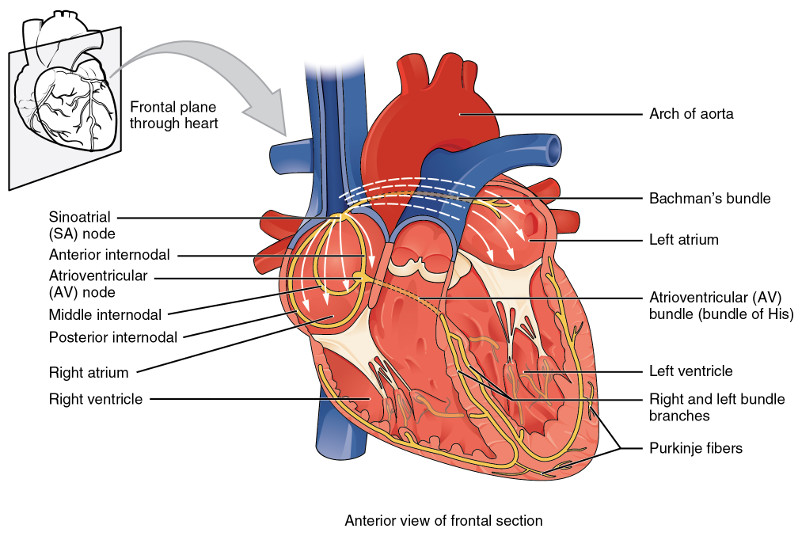
Conduction system of the heart, from Openstax College
Heart beat is caused by the generation of an action potential in the SAN.
The initial increase of the SAN membrane potential from its minimum, rest potential, is due mostly to leakage channels which allow some Na+ to enter the heart, gradually raising the membrane potential from about -60 mV to -40 mV (the “prepotential” in the figure). This is the threshold value for a gated channel to open, allowing depolarization of the cell. However, because of the leakage, only slow Na+–Ca++ channels are opened, which explains the relatively slow rise of the potential. (The slow rate of potential rise from -40 mv to 10 mv is not obvious in the figure.)
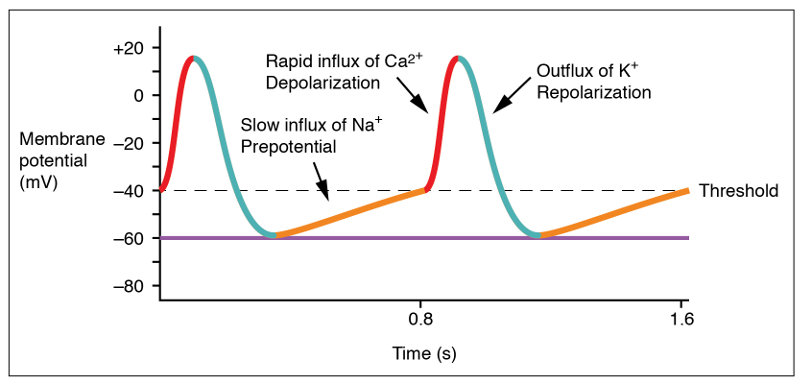
Action potential at the SA node, from Openstax College
After about 100-150 milliseconds, the Na+–Ca++ channels close and K+ channels open. As K+ rushes out of the cell, the potential redescends to a bit under its initial resting value. Then the K+ channels also close and the cycle starts over. The result is that the heart beats all by itself due to the membrane potential of the cardiac muscle cells, the slow leakage of Na+ and the voltage-gated Na+–Ca++ and K+ channels.
The action potential generated in the SAN passes into atrial muscle fibers which contract and squeeze the atria. The action potential travels through the atria and down to the atrioventricular node (AV node), near the floor of the right atrium. A delay takes place here which keeps the ventricles from contracting at the same time as the atria. The action potential then passes into the bundle of His, which separates into two different paths, the left and right bundle branches, which lead to the Purkinje fibers wrapped around the ventricles.
So far, we have only talked about the form of an action potential in the conducting cells. When it reaches the contracting cells, which actually make the muscles squeeze the blood along its way, the form of the action potential is different.
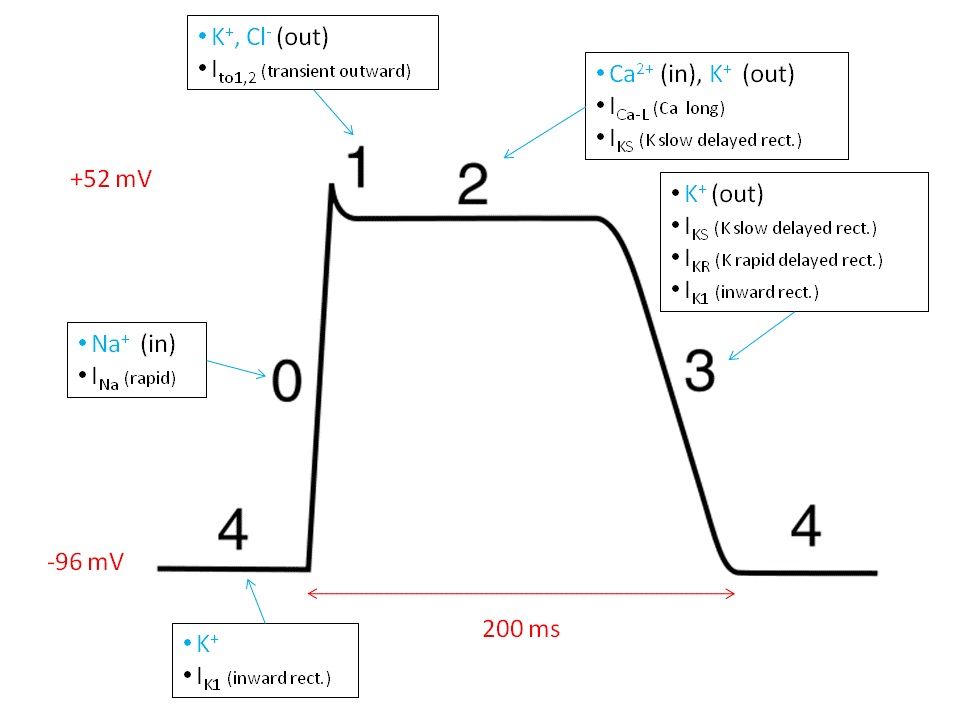
Ventricular action potential, by “Sylvia3” via Wikimedia Commons
The ventricular contracting action potential is shown in the above figure. Since resting ventricular potential is around -90 mV, the fast Na+ channel opens to initiate a fast depolarization. Then the fast channel closes but the slow Na+–Ca++ channels open, maintaining the potential at a plateau until they close and the K+ channels open, repolarizing the cell. This long plateau, or refractory period, of about 200 ms allows time for blood to flow and avoids premature contractions which would disrupt the synchronism of the contractions.
The cardiac cycle
The heart’s electrical signals can be detected on the surface of the skin by an electro-cardiogram (ECG or sometimes EKG) and show the patterns of atrial and ventricular systole (contraction to pump blood out of the heart) and diastole (relaxation while the chambers refill with blood). The output shows cycles of the heart’s functioning over several steps, called the cardiac cycle. It goes like this:
- All chambers are relaxed (diastole) and blood flows into them, filling them to about 70-80% of their capacity. Valves keep the blood from flowing in the wrong direction.
- Depolarization in the SA node starts a series of action potentials which cause atrial contraction (systole) which forces the rest of the blood into the ventricles, filling them completely. This is shown as what is called the P wave on the ECG output. The signal moves down to the AV node, where there is a delay. After about 100ms,the atria relax and enter their diastole phase. They have now completed their activity for this cycle.
- The action potential moves down through the bundle branches and through the Purkinje fibers, launching ventrical systole. The signal reaches the right ventricle first, so it starts to contract slightly before the left. The increased pressure closes the tricuspid and mitral valves to the atria and opens the pulmonary and aortic exit valves (semilunar valves). Blood flows out to the lungs and the body. This stage shows up on the ECG as the QRS complex and lasts about 270ms. Blocks in the branches which impede the signal for whatever reason alter the form of the QRS signal.
- The ventricles relax into diastole at the T wave. Ventrical diastole lasts about 430ms.
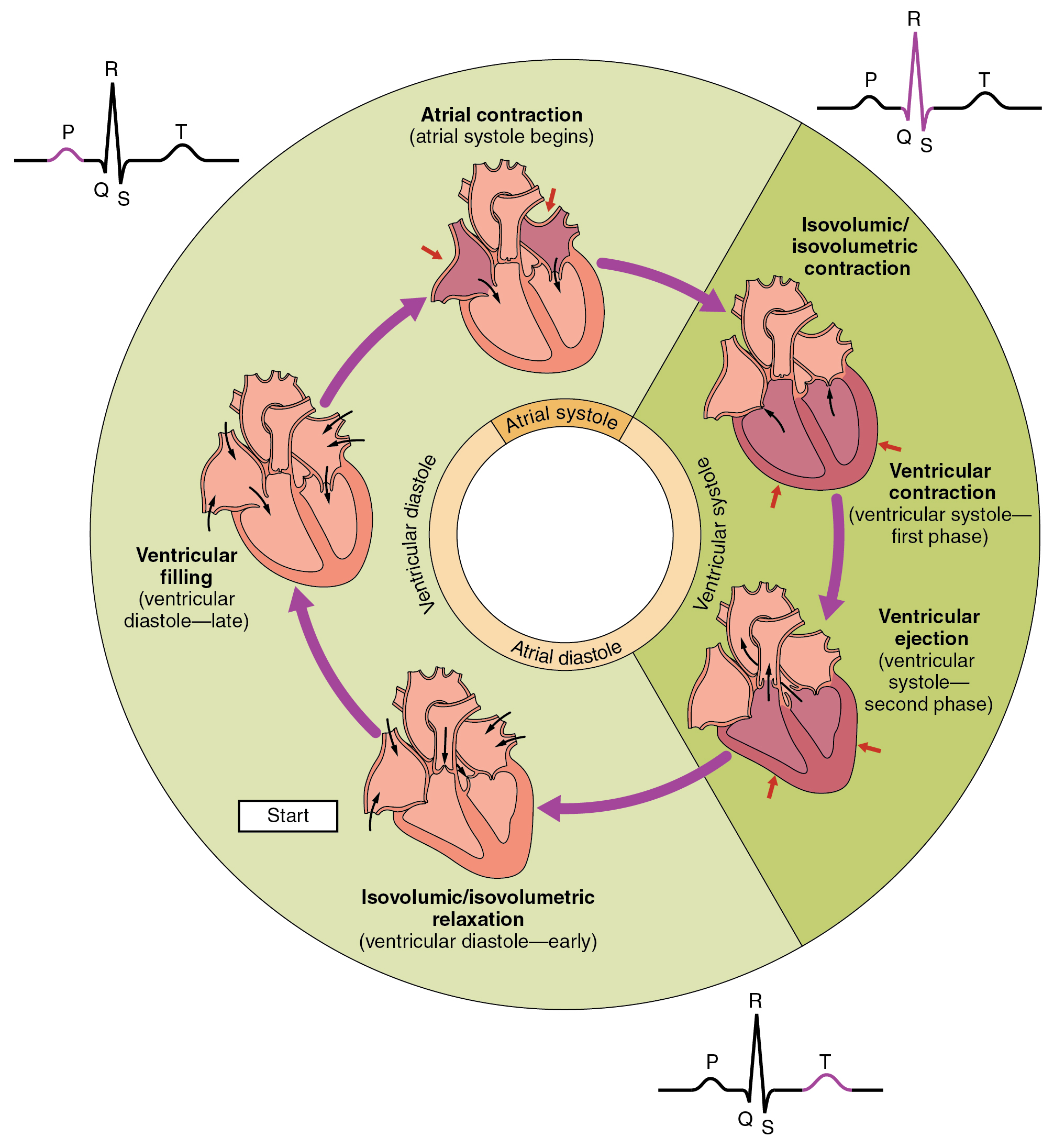
Phases of the cardiac cycle, from Openstax College
The cycle starts over – constantly, all the time we are alive, from our heart’s formation during embryonic development to our last moment of life.
The “lub-dub” sound of a heartbeat, familiar to doctors with stethoscopes and to horror-movie fans, corresponds to the opening and closing of the semilunar (aortic and pulmonary) valves and so to the beginning and the end of the ventricular systole.
Regulation of heart rate
Adding up the durations of the phases indicated above gives 100+270+430 = 800ms per heartbeat, or 75bpm (beats per minute), the average rate for an adult. Although the heart itself is responsible for its beating, both the heart contraction rate (HR) and the volume of the chambers (stroke volume, SV) are influenced in several ways.
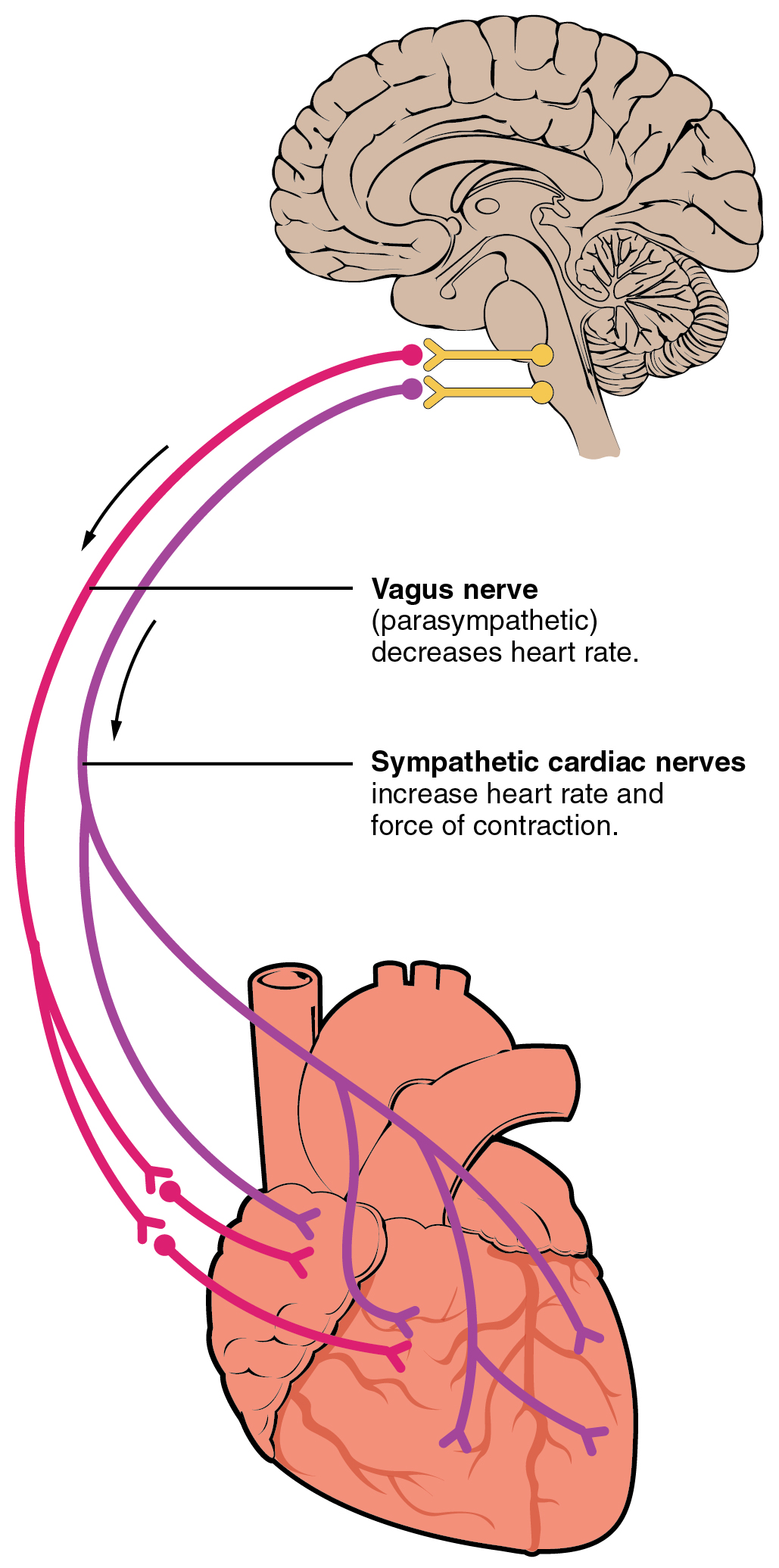
Autonomic innervation of the heart, from Openstax College
Nervous system influence on the heart originates in cardiac centers of the medulla oblongata of the hindbrain, just in front of the cerebellum. Nerve fibers from this region reach several parts of the heart, including the SA and AV nodes and both atria and ventricles. The sympathetic nervous system (e.g., “fight or flight”) releases norepinephrine (NE) which speeds up the heart rate. The parasympathetic system sends acetylcholine (Ach) along the vagus nerve which slows the heart rate. At rest, both (but mostly the parasympathetic) contribute to autonomic tone, which would otherwise be about 100bpm.
Increased physical activity detected by proprioreceptors, changes in blood pressure or flow detected by barioreceptors (stretch) or metabolic activity detected by chemoreceptors send data to the cardiac centers which then regulate heart beat and flow appropriately. Hormones, ion concentrations, temperature, pH and stress (via the limbic system) all have an influence.
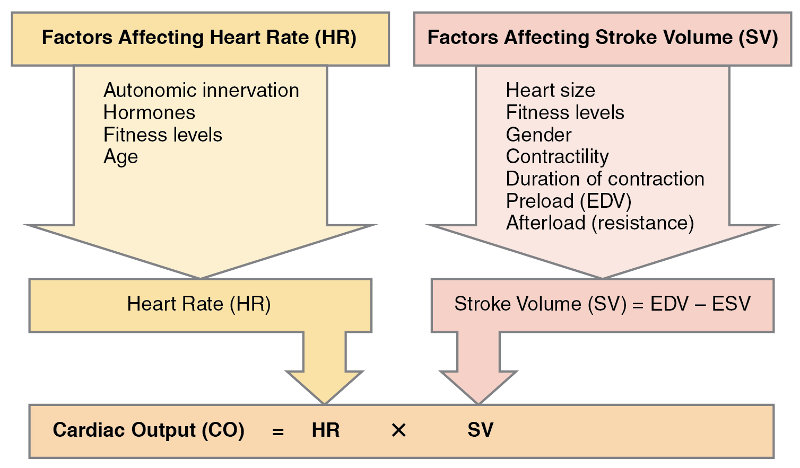
Major factors influencing cardiac output, from Openstax College
Bear in mind that the heart is a muscle and, like the other muscles, needs regular exercise.
The blood
The production of blood and lymphatic system cells from hematopoietic stem cells is shown in the section on the immune system. However, the blood contains other components, listed in the table in the following figure.
Biologists distinguish four types of tissue:
- epithelial tissue (epithelium) covers surfaces, inside the body and out;
- connective tissue provides binding, support, protection and integration;2To my mind, connective tissue is “all the rest”.
- muscle tissue contracts and consists of three types: skeletal (voluntary), smooth and cardiac;
- nervous tissue propagates electrochemical signals.
Blood is considered to be connective tissue.3Obviously, it is not one of the others. Connective tissue consists of cells which are relatively far apart, compared to epithelial cells, and which are dispersed within a matrix, usually a liquid. The cells of blood, called the formed elements, float in an extracellular matrix called plasma, which is mostly water. The components of blood are shown in the following table.
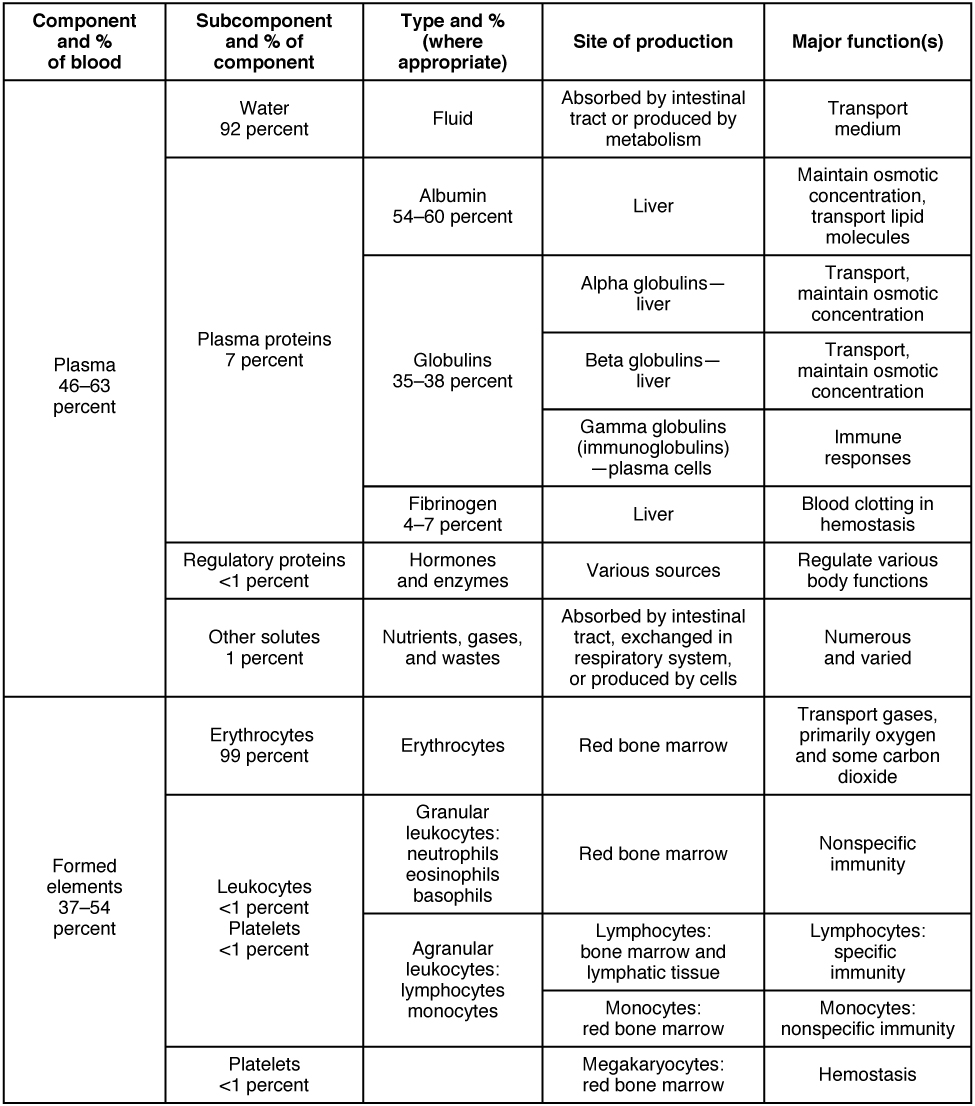
Major blood components, from Openstax College
Although blood plasma is about 92% water, it does contain three types of plasma proteins as well as small amounts of other substances.
Aside from water, the major components of the plasma are albumin and and globulins. Albumin binds to lipids and transports them through the hydrophilic plasma. It also serves to regulate osmotic pressure. Globulins, or gamma globulins, are immunoglobins, i.e., antibodies, about which more shortly.
Blood serves many purposes, but the most important (although it could not go on without the others) is the transport of oxygen, nutrients and waste matter, such as carbon dioxide.
The transport of oxygen is carried out by erythrocytes, or red blood cells (RBC), which make up 99% of the formed elements. They are so numerous, they make up approximately 25% by number of all the cells in the body.
As an erythrocyte develops in the marrow of large bones, it ejects most of its organelles, including the nucleus and the mitochondria. Without mitochondria, it is only capable of anaerobic respiration, which prevents its using up the very oxygen it is transporting. Erythrocytes are shaped like tiny disks, thinner in the middle, which enables them to fold up to fit through small openings in capillary walls. They are extraordinarily well adapted to their function. For instance, the absence of many internal organelles leaves more space for the molecule which transports oxygen: hemoglobin.
A molecule of hemoglobin is made up of four folded protein chains called globin, each one of these attached to a red pigment molecule called heme, which contains one ion of iron, Fe2+. To each iron ion, one molecule of oxygen can bind for transport, making four oxygen molecules per hemoglobin molecule. The result is called oxyhemoglobin and constitutes the bright red blood which flows in non-pulmonary arteries and pulmonary veins. The oxygen-transporting capacities of the body’s hemoglobin are quite phenomenal. One erythrocyte contains about 300 million hemoglobin molecules and so binds up to 1.2 billion oxygen molecules.
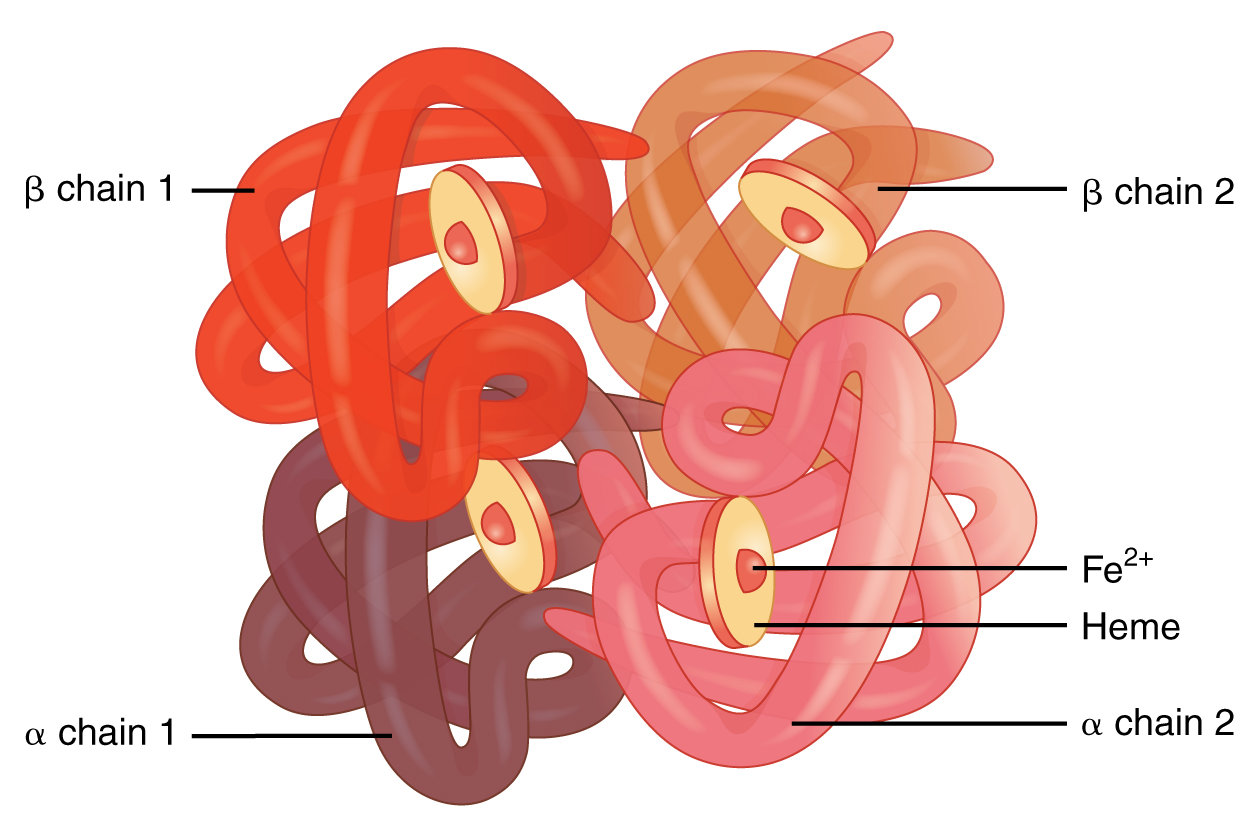
Hemoglobin molecule, modified after Openstax College
Even so, there are not too many. If the number goes down, we suffer from anemia, if not something worse. The composition of hemoglobin underlines the necessity of small amounts of trace elements like iron in the body.
The amount of oxyhemoglobin is in equilibrium with the milieu:
- A higher concentration of oxygen leads to a greater amount of oxyhemoglobin formed;
- a lower concentration of oxygen causes oxyhemoglobin to dissociate into oxygen and hemoglobin.
This explains why oxyhemoglobin forms in the oxygen-rich lungs and dissociates in the capillaries where oxygen is less concentrated. In addition, the presence of CO2 in the blood somewhat increases the dissociation of oxyhemoglobin.
Next, the lymphatic system.