Our nutriments are composed almost entirely of three types of substances – carbohydrates (glucids, in some countries), proteins and lipids (or fats); the rest consists of small amounts of minerals and vitamins (organic nutrients required in small amounts, but which the organism cannot synthesize). The sequences of biochemical processes which constitute use of these three types of molecules are called metabolic pathways.
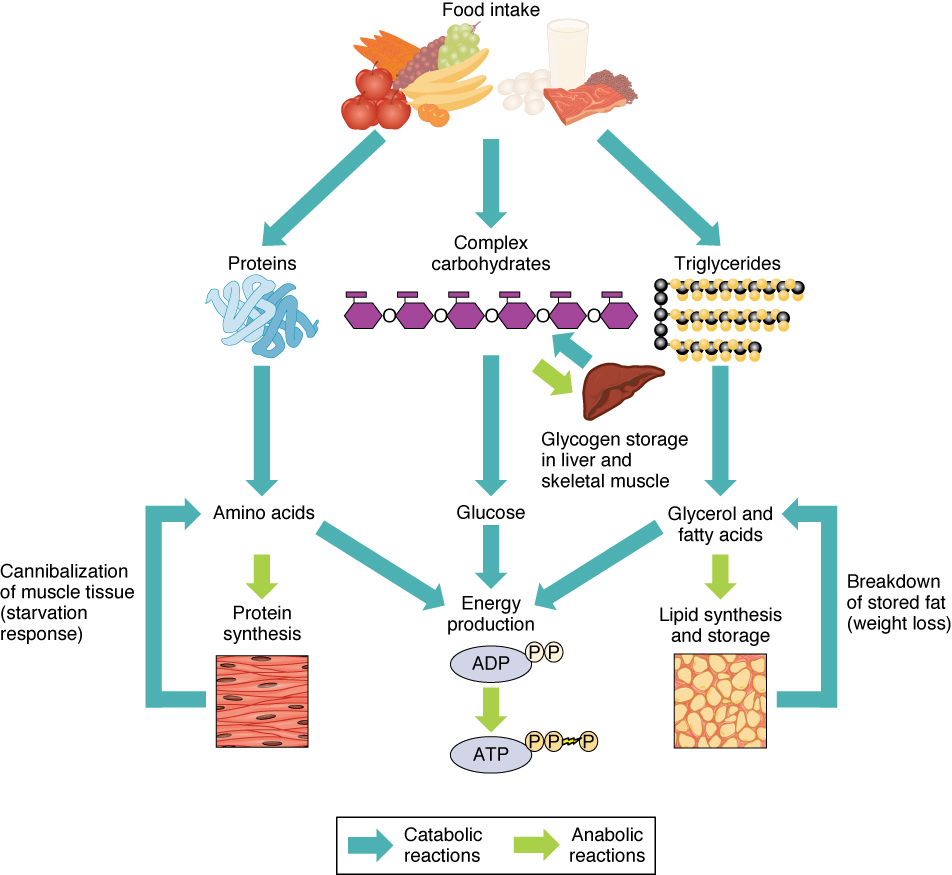
Sources of ATP, from Openstax College
The very notion of metabolic pathways is somewhat of an idealization, since science is not that simple when we look closely. In fact, as the image shows, the pathways are connected at multiple points. In addition, there are inputs of enzymes and hormones from other organs.
In addition, there are inputs of enzymes and hormones from other organs. The liver is essential in energy regulation and storage and produces bile, which is needed for digestion of fats and lipids. Bile can be stored in the gallbladder to be delivered to the duodenum (the first part of the small intestine) when needed. The pancreas secretes enzymes essential for the digestion of all three food types.
Digestion in the small intestine is similar for all three principal food types. Carbohydrates, fats and proteins are all broken down by hydrolysis, adding a water molecule to separate the long molecules into shorter ones. For a simple disaccharide represented by R’’-R, this can be represented chemically by:
R’’-R’ +H2O → R’’OH + R’H,
where the hydrolysis process indicated by the arrow requires the use of an enzyme.
Basic digestion of the three food types goes like this:
- Carbohydrates are composed of poly- and monosaccharides. These are reduced by digestion to monosaccharides, which are used for energy or stored in cells, especially liver and muscle cells.
- Proteins are polypeptide chains of amino acids, which are broken down into the separate amino acids which will be used to make more proteins.
- Fats are composed mainly of triglycerides, which are separated into fatty acids.
Different enzymes are used in the three case.
The following sections discuss digestion of each type in more detail.1Principal source of information, Guyton and Hall (2011), chaps. 67-69.
Digestion of carbohydrates
Carbohydrates are composed mainly of sucrose, lactose, long-chain starches and cellulose. Cellulose is not digestible and just passes through the body in the form of fibers that nutritionists are so fond of.
Food is first ground by the teeth, which facilitates subsequent digestion. Ptyalin, a digestive enzyme found in saliva, begins hydrolysis. Only about 5% of hydrolysis takes place in the mouth before the food is moved by the tongue into the pharynx (throat). The epiglottis ensures that it moves past the tracheal opening and into the esophagus (a process which sometimes functions less well in older people), through which it descends into the stomach. Hydrolysis continues there until the food becomes mixed with enough gastric secretions that the mounting acidity renders the enzyme inactive. After being kneaded in acidic gastric juice, the resulting pasty chyme passes into the small intestine, which secretes mucus, hormones and digestive enzymes.
By the time food enters the small intestine, 40-50% of carbohydrates have been hydrolyzed, principally to maltose. Further digestion of carbohydrates takes place in the small intestine as pancreatic amylase, an enzyme secreted by the pancreas, breaks them down into maltose or small glucose polymers, large molecules composed of repeated subunits. Different enzymes then split these into their constituent monosaccharides, the simplest forms of carbohydrates, which are soluble and so can cross the intestinal wall to enter the blood stream and be carried to the liver.
- Maltose from starches is broken down by maltase and a-dextrinase to glucose.
- Lactose from milk is converted by lactase into glucose and galactose.
- Sucrose (table sugar) is converted by sucrase into fructose.
The products are therefore glucose (about 80%), fructose and galactose. The last two substances are converted by the liver into glucose, which is therefore the common final form of carbohydrates arriving in cells. It is either used right away for energy, being sent to the glycolytic pathway and thence to the Krebs cycle, or converted to glycogen (by the process of glycogenesis) and stored.
Glycogen is a large polymer of glucose molecules and is an efficient form for storage. Storing glycogen instead of the individual glucose molecules allows more storage without substantial modification of the cell’s osmotic pressure.2Since osmotic pressure depends on the number of molecules in a solvent. See the discussion of osmosis and related possible danger for cells in the section on water. All the body’s cells can store small amounts of glycogen, but the champions are the liver (5-8% of its weight) and the muscles (1-3%).
When the body’s glycogen storage capacity is filled, excess glucose is converted to acetyl-CoA and thence into triglycerides (lipids), which are stored in adipose tissues. Yes, too much sugar turns to fat. Fat contains ~2.5 times the energy of an equal weight of glycogen. So since much more fat than glycogen can be stored in the body, an average person stores around 150 times as much energy in fat as in carbohydrate.
Digestion of proteins
Proteins are partially broken down in the stomach by the enzyme pepsin, important because it is capable of breaking down the protein collagen in the connective tissue of meat. This enzyme prefers a pH of 2.0-3.0, which is maintained by hydrochloric acid secreted at pH 0.8 by gastric glands in the stomach. When the resulting chyme passes into the small intestine, the pancreas excretes sodium bicarbonate to lower the acidity. As in the case of carbohydrates, the pancreas contributes enzymes, pancreatic proteolytic enzymes or proteases, to the small intestine to continue the breakdown of polypeptides into amino acids, although most remain as relatively small di- or tripeptides. Finally, inside the cytosol of enterocytes3Columnar epithelial cells, i.e., cylindrical cells on the intestinal wall, whose large surface area facilitates the transfer of molecules from the intestine. on the intestinal wall, various peptidases break down what is left into single amino acids which then pass into the blood. These are carried to the cells to be used as raw materials for the construction of new proteins in ribosomes. Any remaining peptides may cause serious problems.
In order to prevent proteolytic enzymes from destroying needed bodily proteins, they exist in unactivated precursor forms called zymogens and are only turned on when needed.
Digestion of lipids
Lipids (fats) consist of triglycerides, phospholipids, cholesterol and small quantities of other substances. Lipids are necessary for the formation of cell membranes and for many functions within cells. Like carbohydrates, they can provide energy. Most ingested fats are neutral fats called triglycerides, consisting of a glycerol nucleus attached to the ends of three fatty-acid side chains. Phospholipids are composed of only two fatty acids, attached to a hydrophilic phosphate “head group”.4This subject was discussed in some detail in a preceding section. Cholesterol is not a fat but has chemical properties similar to those of fatty acids and is digested similarly,
Fat digestion occurs mainly in the small intestine. The problem is that fats are not soluble in water. The hydrophobic fat globules are relatively large and must be broken up by emulsification before they can be effectively acted upon by enzymes. First, fat is mixed with bile (bile salts and, especially, lecithin) with polar and non-polar parts. The non-polar parts attach to the fats, leaving their hydrophilic polar parts pointing outwards. When they are agitated, they break up to form an emulsion, a mixture of one normally non-miscible liquid in another (like well-stirred vinaigrette salad dressing, or detergent in dish water). The smaller units of the fat present a much greater surface area than before and so can be attacked by water-soluble pancreatic lipase and split into free fatty acids (FFAs) and monoglycerides (the glycerol and the attached middle fatty acid ).
Again, the products are surrounded by bile salts with their non-polar ends towards the fats and their polar ends pointing outwards into the water. They then form small spheres called micelles, globs of which are water-soluble. The micelles then ferry the FFAs and monoglycerides through the aqueous solution to the epithelial cells. Since these are also fatty, the lipids can diffuse into them, leaving the bile behind behind to form more micelles.
The same processes of hydrolization and ferrying by micelles takes place for phospholipids and cholesterol using different pancreatic lipases.
Inside the intestinal membrane, the FFAs are re-synthesized into triglycerides. The triglycerides cholesterol, phospholipids and some apoprotein B (about 9% phospholipids, 3% cholesterol and 1% apoprotein B) are packaged into tiny (0.08-0.6 microns) phospholipid vesicles called chylomicrons whic leave the intestinal cells. They are too big for capillaries but can flow via lacteals into the lymphatic system.5See the chapter on the lymphatic system. The chylomicrons transport the fats and cholesterol through the aqueous lymph and then into the venous blood via the thoracic duct. From the blood, they are stored either in the liver or in adipose tissue.
Triglycerides are converted into FFAs and back because triglycerides cannot cross the intestinal cell membranes, but FFAs can. They then enter the lymphatic system. Only small amounts of water-soluble, short-chain fatty acids like butterfat can pass directly from the small intestine into the blood without making a detour through the lymphatic system.
In short, fats are emulsified by bile salts so that they can be broken up by pancreatic lipase into FFAs. FFAs can cross the intestinal membrane and are reconverted to triglycerides. In order for them to be transported in the aqueous lymphatic system, FFAs and cholesterol are enclosed in phospholipid vesicles, called chylomicrons.
In this manner, almost all ingested fats (except short-chain fatty acids) are absorbed from the intestine into the intestinal lymphatic system in chylomicrons. These then are passed through the thoracic duct into venous blood. Several types of tissues, especially skeletal muscles, adipose tissues and heart tissues, synthesize lipoprotein lipase which hydrolyzes the triglycerides from the chylomicrons. The resulting fatty acids are either used for energy or stored in the cells until needed.
After breakup of the chylomicrons and their removal by the liver, most of the lipids left in blood plasma (>95%) are in the form of tiny particles called lipoproteins, partly lipid and partly protein, which are like miniature chylomicrons. The lipoproteins may contain triglycerides, phospholipids, cholesterol or proteins. Like chylomicrons, lipoproteins serve to transport lipids in the blood. Originally, use of a centrifuge to separate their components led to the definition of four classes of lipoproteins, based on their densities
In the body, though, no centrifuge exists to distinguish among the different densities of lipoproteins. Lipoproteins sent out by the liver carry triglycerides and are referred to as VLDLs (very-low density lipoproteins), since triglycerides are lighter than water. As tissues absorb the triglycerides, the lipoproteins become denser, effectively becoming IDLs (intermediate=density) and then LDLs (low-density). LDLs serve essentially to deliver cholesterol to tissues. But HDLs (high-density) are made up of different proteins. Their function is to carry cholesterol away from tissues and back to the liver.1 Hence, the slightly incorrect practice of calling LDLs “bad cholesterol” and HDSs “good cholesterol”.
The fat intake of the world’s peoples is quite variable, being 10-15% in parts of Asia and 35-50% in western countries. Part of this is converted to triglycerides and stored.
Use of fats for energy — energy production from metabolic pathways
Compared to carbohydrates and proteins, triglycerides furnish more than twice the energy per unit mass. So when glucose levels become inadequate, stored triglycerides are hydrolyzed to fatty acids. The rate of conversion and transport of FFAs is great enough that they can be oxidized to fulfill almost all the body’s energy needs without the need of energy from carbohydrates. Most cells except brain tissue and red blood cells can get their energy from fatty acids.
In order to be used for energy, triglycerides are converted by lipolysis to glycerol and fatty acyl CoA, the latter carried by carnitine into the mitochondria of the cells. There, it is converted back to fatty acids, then degraded by the process of beta-oxidation into acetyl-CoA, which enters the Krebs cycle of cellular respiration, the products of which also feed the electron transport chain (explained shortly).
Glycerol is converted directly into a form which can enter the glycolytic pathway (glycolysis, the introductory step to the Krebs cycle).
glycerol → glycero-3-phosphate → glycolytic pathway → energy
The liver stores a large quantity of fatty acids, but uses only a small part of these for its own energy requirements. So when carbohydrate stores (including glycogen) are low, great use is made of fats for energy. But, due to lack of intermediary substances, the Krebs cycle slows down. Excess acetyl-CoA accumulates in the liver where it is combined in pairs to form acetoacetic acid which is then carried by the blood to other cells. Some of it is converted to β-hydroxybutyrate and acetone, large quantities of which can be transported rapidly to cells.6Differences between Guyton and Hall and Openstax about the order of these two processes. Which came first, acetoacetic acid or β-hydroxybutyrate and acetone? The end result is the same. These two substances plus acetoacetic acid are collectively referred to as ketone bodies and their synthesis from fats is called ketogenesis. On arriving in cells, all three are converted back into acetyl-CoA, which enters the Krebs cycle. This Is interesting because, in such circumstances, the brain will be lacking energy because fats can not cross the blood-brain barrier to supply it. But ketone bodies can. So the brain goes straight from glucose input to ketone bodies without ever using fats.
In brief, fats stored as triglycerides in the liver may be modified to ketone bodies and enter cells where they are transformed into acetyl-CoA and enter the Krebs cycle even in the brain.7See the figure, “The steps of cellular respiration”, in chapter “Making energy available“.
The preferred order of nutrients for energy is simple: Carbohydrates come first. But even the glycogen stored mainly in the liver can only furnish the body in fuel for maybe a half day. If carbohydrates run out, fats and proteins start to be used, principally fats. Use of fats continues approximately linearly until they run out. At the same time, stored amino acids are converted by gluconeogenesis to glucose, which is used. When the easily-accessed amino-acid store is reduced, fat usage increases and some of this is converted to ketone bodies, which can enter the brain and supply energy to it. However, changing from a carbohydrate-rich to a fat diet may require several weeks for brain cells to switch (mostly) to energy from fats.8Guyton and Hall, 2011, p. 824.
Regulation of glucose levels
The liver converts glucose into glycogen and stores that; it can be reconverted whenever the level of blood sugar becomes low. This function is mediated by the hormones insulin and glucagon, both produced by the pancreas but having opposite effects.
Glucose concentration in the blood needs to be within a certain limited range in order to distribute enough energy to the body, but not so much as would harm organs and tissues, especially where blood vessels are tiny, as in the retina, the body’s extremities and the kidneys. Most cells have receptors which bind insulin, causing glucose transporters in the cell membranes to allow more glucose to enter the cell for storage, thereby reducing the glucose level of the blood. Abnormally high glucose levels in the blood are a sign of diabetes, which can be due to inadequate insulin production or to the cells’ not reacting correctly to insulin presence.
If the blood glucose level becomes too low, the pancreas secretes glucagon which stimulates production of glucose from the breakdown of glycogen, from amino acids or from stored triglycerides (lipolysis).
Now all this energy must be made available by the process of cellular respiration.
Notes
↑1 | Principal source of information, Guyton and Hall (2011), chaps. 67-69. |
---|---|
↑2 | Since osmotic pressure depends on the number of molecules in a solvent. See the discussion of osmosis and related possible danger for cells in the section on water. |
↑3 | Columnar epithelial cells, i.e., cylindrical cells on the intestinal wall, whose large surface area facilitates the transfer of molecules from the intestine. |
↑4 | This subject was discussed in some detail in a preceding section. |
↑5 | See the chapter on the lymphatic system. |
↑6 | Differences between Guyton and Hall and Openstax about the order of these two processes. Which came first, acetoacetic acid or β-hydroxybutyrate and acetone? The end result is the same. |
↑7 | See the figure, “The steps of cellular respiration”, in chapter “Making energy available“. |
↑8 | Guyton and Hall, 2011, p. 824. |