Overall brain structure and evolution
The human brain weighs about 1.5 kg and contains on the order of 100 billion (1011) neurons, each with an average of perhaps a thousand connections. The brain also includes the retinas of the eyes and extends down to the spinal column. It consists of an inner and evolutionarily older part which is covered over by the cortex (or neocortex, if you consider the older part as the mesocortex). The neocortex is about 2500 cm2 (50 cm on a side) if spread out, but is compressed like a wadded-up piece paper so it contains creases called sulci (singular, sulcus) and bumps called gyri (singular, gyrus).
Over the last two million years of evolution, the size of the hominid brain has almost tripled. The brain has not just grown, it has changed its form. There are no direct fossil records of brains, only skeletal craniums which may indicate brain size and, sometimes, the shape of the cortex. It seems that as hominid brains increased in size, the cortex grew relatively larger and was reorganized in a way that facilitates emerging cognitive abilities, e.g., language. All this is the subject of paleoneurology, a whole domain in itself and beyond the scope of our considerations.
Following in the footsteps of geologists and biologists who learn about the past by studying the present, we can deduce Information about evolution by comparing the brains of different contemporary animals. The less-developed brains of animals like lizards possess only some of the components found in mammal brains. In particular, the neocortex which covers all the rest of the mammal brain is non-existent or much more rudimentary in “lower” animals such as reptiles. Hence, the lower part of the human brain has been called the “reptile brain”. That is not to say it does not have essential functions, because it certainly does.
The brain contains a fairly large number of functional elements. Today, brain structures are grouped according to embryonic development of the brain, as already presented on the page about developmental biology. This has not always been the case; terms like “limbic system” remain in use, sometimes leading to confusion. The following figure recalls the developmental parts of the brain.
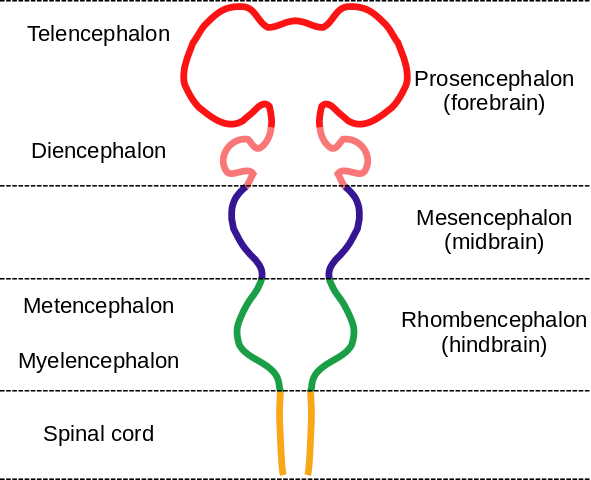
Embryonic brain parts, by “Nrest” via Wikimedia Commons
Cellular components of the brain
Neurons
Neurons are cells and so share the properties of cells, including membrane potentials and organelles, discussed in preceding chapters.
A neuron possesses a cell body and various organelles, including a nucleus and mitochondria, like almost any other eukaryotic cell. In addition, a neuron possesses a “tree” of input branches called dendrites and generally one long output branch called an axon, usually terminating in a number of smaller axon branches. Dendrites and axons together are referred to as neurites. Chemical signals called neurotransmitters are passed from the axon terminal of one neuron across the gap, called a synapse, separating it from another neuron’s dendrite terminal. If the second neuron receives a sufficiently great chemical signal, an action potential is created (as explained previously) which passes down through the cell’s axon and out to the synapse to another neuron, where a chemical signal again is released. This process is then repeated. Through multiple dendrite and axon terminals, one neuron can communicate with many others to form a network. This is basis of neuronal function.
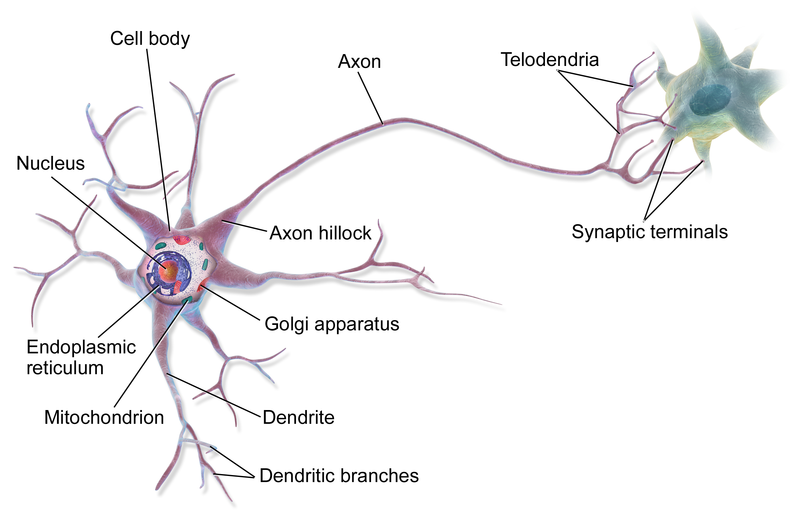
A multipolar neuron, by BruceBlaus via Wikemedia Commons
Neuroscientists classify neurons in different ways.
The simplest distinction simply classes neurons by their number of neurites: unipolar, if one; bipolar, if two; multipolar, if more than two, like the above. In the cerebral cortex, the two principle types of neurons, as defined by the structure of their neurite trees, are pyramidal (triangular) cells, with one principal neurite running toward the outer and one toward the inner side of the cortex, and stellate cells, with many projecting neurites. Dendrites may be spiny (complicated) or not (aspinous). Neurons may be projection neurons (Golgi type I), projecting to farther parts of the brain, or local circuit neurons (Golgi type II), sticking around home. Pyramidal cells tend to have long dendritic trees and so are projection neurons.
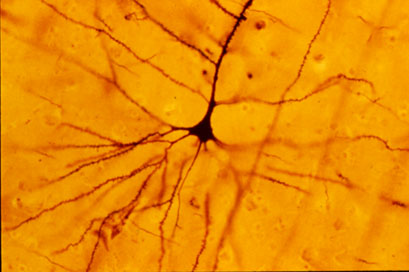
Pyramidal cell, from Wikimedia Commons
Neurons are also classified according to the neurotransmitter they use. For instance, cholinergic neurons use the neurotransmitter acetylcholine.
Our senses depend on detecting objects in the external world and they use receptors to do this. A receptor is generally a gated receptor, meaning that some event causes the receptor to open an ion channel. Here are some examples of such events:
- light energy (photons) on the photoreceptors of the retina;
- sound energy (pressure waves in the air) on the ear drum and the auditory cilia;
- stretching of the skin detected by somatosensory mechanoreceptors in the dermis;
- chemical substances on olfactory receptor cilia in the nose or on the taste buds of the tongue;
- binding of a specific substance (neurotransmitter) to the exterior part of the receptor ion channel (in communication between neurons);
- electric potential on voltage-dependent sodium channels (leading to depolarization of the cell).
Some neurons transmit output to the motor system, where different axon terminals activate sets of muscle fibers called motor units.
In neurons, a signal is generated when a neurotransmitter molecule stimulates a ligand-gated channel specific to that neurotransmitter. The neurotransmitter may be excitatory or inhibitory.
- An excitatory neurotransmitter contributes to forming an action potential by depolarizing the cell, as already described.
- An inhibitory neurotransmitter opens either a Cl- or a K+ channel. Either Cl- flowing into the cell or K+ flowing out reduces the membrane potential, which is said to be hyperpolarized, thereby reducing the probability of forming an action potential.
Receptors are also of two types according to their functioning.
- Receptors such as the ligand-gated ion channels we have seen which let an ion pass through a pore in the same protein as the receptor are called ionotropic.
- Some receptors do not open a pore or allow an ion to pass. Instead, when the neurotransmitter, called the first messenger, binds to the extracellular side of the receptor, the intracellular side causes a G protein to activate an effector protein. The effector, which is an enzyme, causes generation of a molecule called a second messenger. The second messenger may bring about other events, often multiple or cascading. Such receptors are called metabotropic. An example of a second messenger is cAMP, which we met in discussion of the lac operon, and which is generated by the effector adenylyl cyclase.
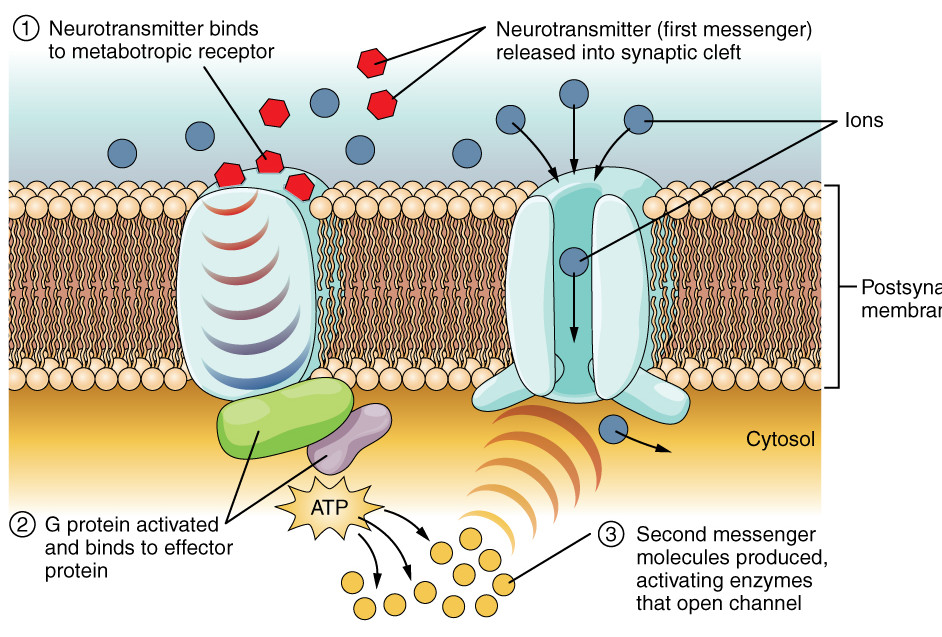
Metabotropic receptor, modified after Openstax College
If the net result is excitatory, an action potential flows down the neuron and along the axon, the output branch. Long axons are usually wrapped in a myelin sheath, which allows the signal to travel much faster than without it. There are breaks in the sheath called nodes of Ranvier where voltage-gated channels are activated to regenerate the action potential, acting as amplifiers of the action potential. Since the action potential jumps from node to node rapidly, this is called saltatory (jumping) conduction.
On arriving at the end of the axon terminal, the action potential activates a voltage-gated channel which allows Ca++ to enter the terminal. These ions cause vesicles containing the appropriate neurotransmitter to fuse with the cell walls and release the neurotransmitter across the post-synaptic cleft and onto the postsynaptic receptor on a dendrite of another neuron. There, the process may start all over again.
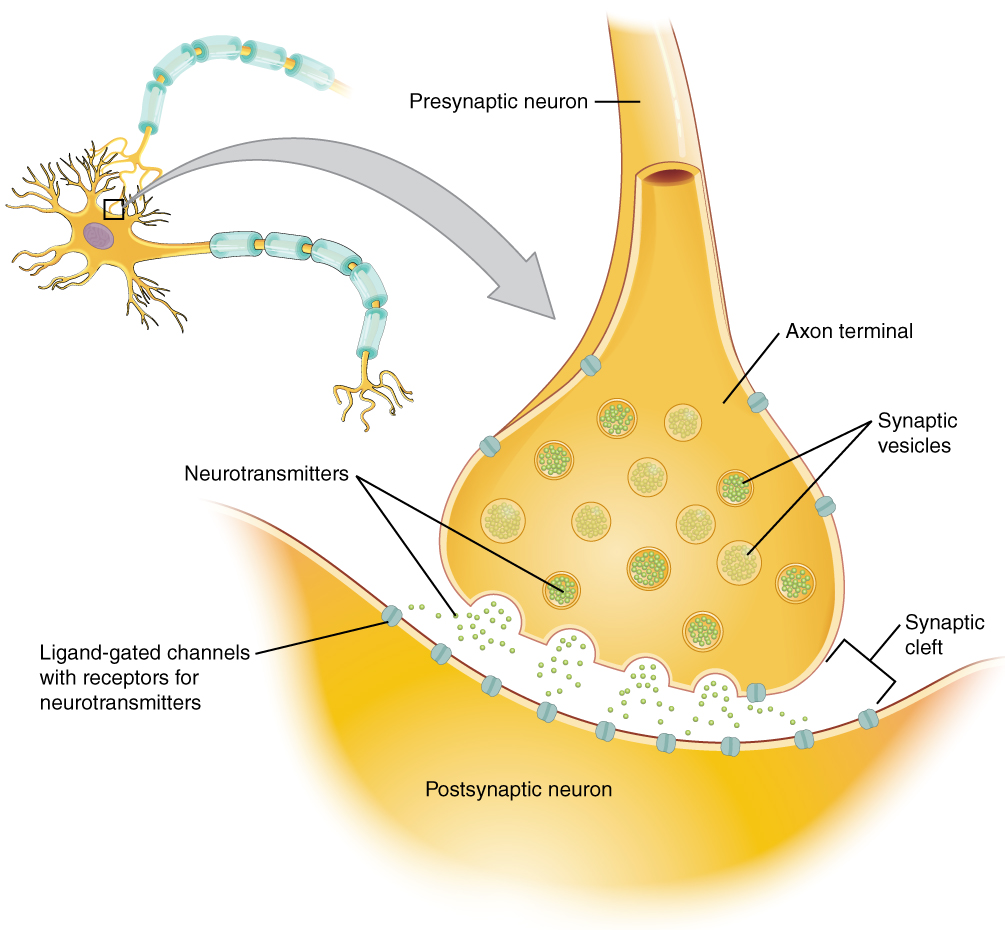
Chemical synapse between neurons, from Openstax College
Quick review:
Voltage-gated channels in the pre-synaptic terminal allow entry of Ca++, which induce release of a chemical neurotransmitter. On arriving at the post-synaptic terminal, the neurotransmitter opens a ligand-gated channel which may induce an electrical action potential. So a pre-synaptic electric signal has been linked to a chemical signal which is converted back into an electric signal in the post-synaptic terminal. Electricity to chemistry and back to electricity. In this way, electrochemical signals are propagated from one neuron to another or others, setting up “currents” of communication in the nervous system. These currents or circuits are the basis of what goes on in the brain – reflexes, emotions, thoughts, planning – the whole shebang.
Glia
As already stated, there are about 100 billion neurons in the brain with an average of 1000 or so synapses each, impressive numbers. But there are something like ten times that many other cells, called glial cells or glia. Glia are essential for the proper functioning of the neurons.
Glia come in six types. The following four are found in the central nervous system (CNS).
- Star-shaped astrocytes form a support system for neurons. They maintain chemical concentrations and are part of the blood-brain barrier (BBB), which uses active-transport mechanisms to limit what molecules can pass from the blood into the CNS. They allow in glucose and amino acids, for instance, but deny passage to many other things, which can be a problem for administering medications to the brain.
- Oligodendrites (with few branches, “oligo” meaning “few”) have processes1Projections of tissue. which surround axons with myelin sheaths.
- Microglia act as macrophages, surrounding and digesting damaged cells or pathogens. They function as the immune system of the brain.
- Ependymal cells line the ventricles of the brain and filter blood to provide cerebrospinal fluid (CSF), which circulates throughout the CNS.
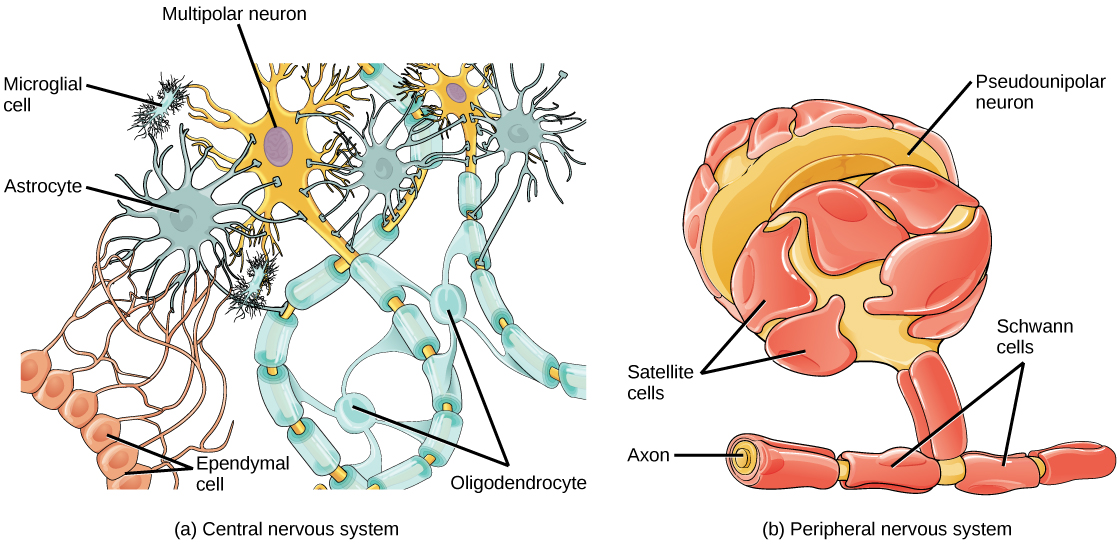
Glial cells, from Openstax College
Two other types of glia are found in the peripheral nervous system (PNS).
- Satellite cells surround cell bodies in ganglia and provide support similarly to that of astrocytes in the CNS.
- Schwann cells are similar to oligodendrites, providing myelin sheaths for axons, but one Schwann cell surrounds only one axon and dos not have multiple processes.
Now let’s go on to look at brain growth and development.
Notes
↑1 | Projections of tissue. |
---|