The discoveries made by geologists and paleontologists about earth’s history make one of the most interesting and illuminating stories ever. That is why so many folk traditions have imagined their own versions of the story in the days before the empirical sciences. Even though the details of some of the mechanisms behind the dynamics of earth science are not yet completely understood, the knowledge gained explains very satisfyingly – and in great detail – how and why the earth and its inhabitants, including humans, have come to be what they are today. It also gives hints as to what may happen tomorrow. So read on.
There is still discussion as to the standard to be used for “million years ago”: “Mya” or “MA”. We will use the more intuitive Mya = million years ago, Gya = billion (thousand million, or giga) years ago. “My” then defines a duration of a million years.
Some geophysics (Earth physics)
First, let us look at the composition of the Earth.
Minerals and rocks
Almost all rocks are made up of minerals, which are naturally occurring, inorganic, crystalline solids. Geologists distinguish them by their color, luster (how they reflect light), transparency (compare diamond and coal), streak (powdered form), hardness, tenacity, and cleavage and fracture (how they chip or break).
The mass of the Earth is constituted of the following proportions of elements by mass1Marshak, 142.:
- 32.1% iron
- 30.1% oxygen
- 15.1% silicon
- 13.9% magnesium
- 8.8% other elements, especially aluminum and calcium.
The most common elements in rocks at the Earth’s surface are silicon and oxygen. These form silicate, a mineral having a tetrahedral structure rather like that of methane, as we saw in the carbon chapter. This image shows two of them bound together by sharing an oxygen between two tetrahedra.
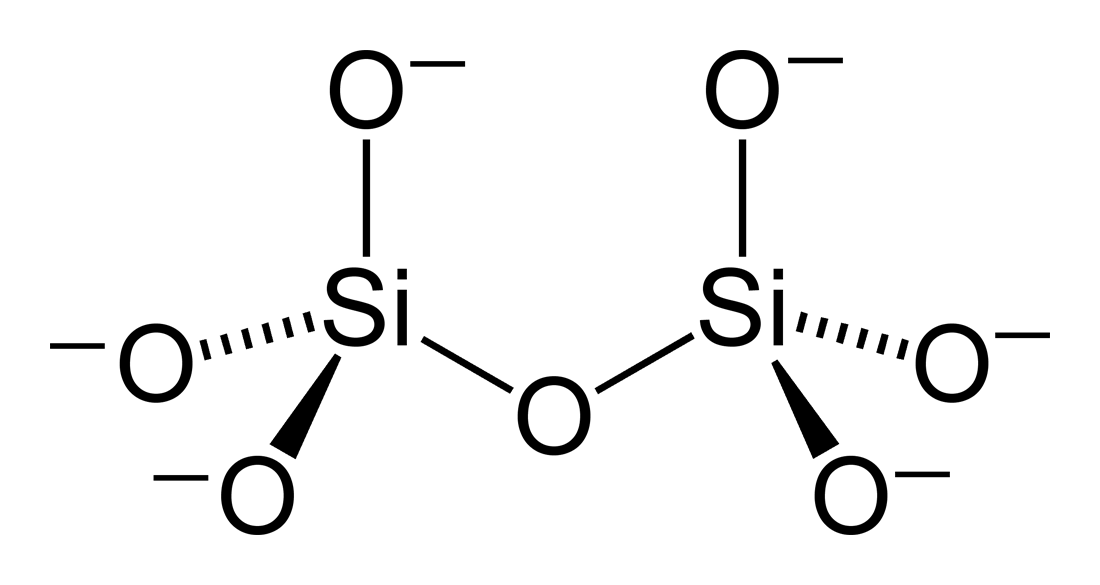
Silicate double tetrahedra, by Ben Mills via Wikimedia Commons
This silicate binding can be extended to give the many different crystalline structures, such as rings and chains, which make up the rocks around us. For instance, quartz crystals have the following structure.
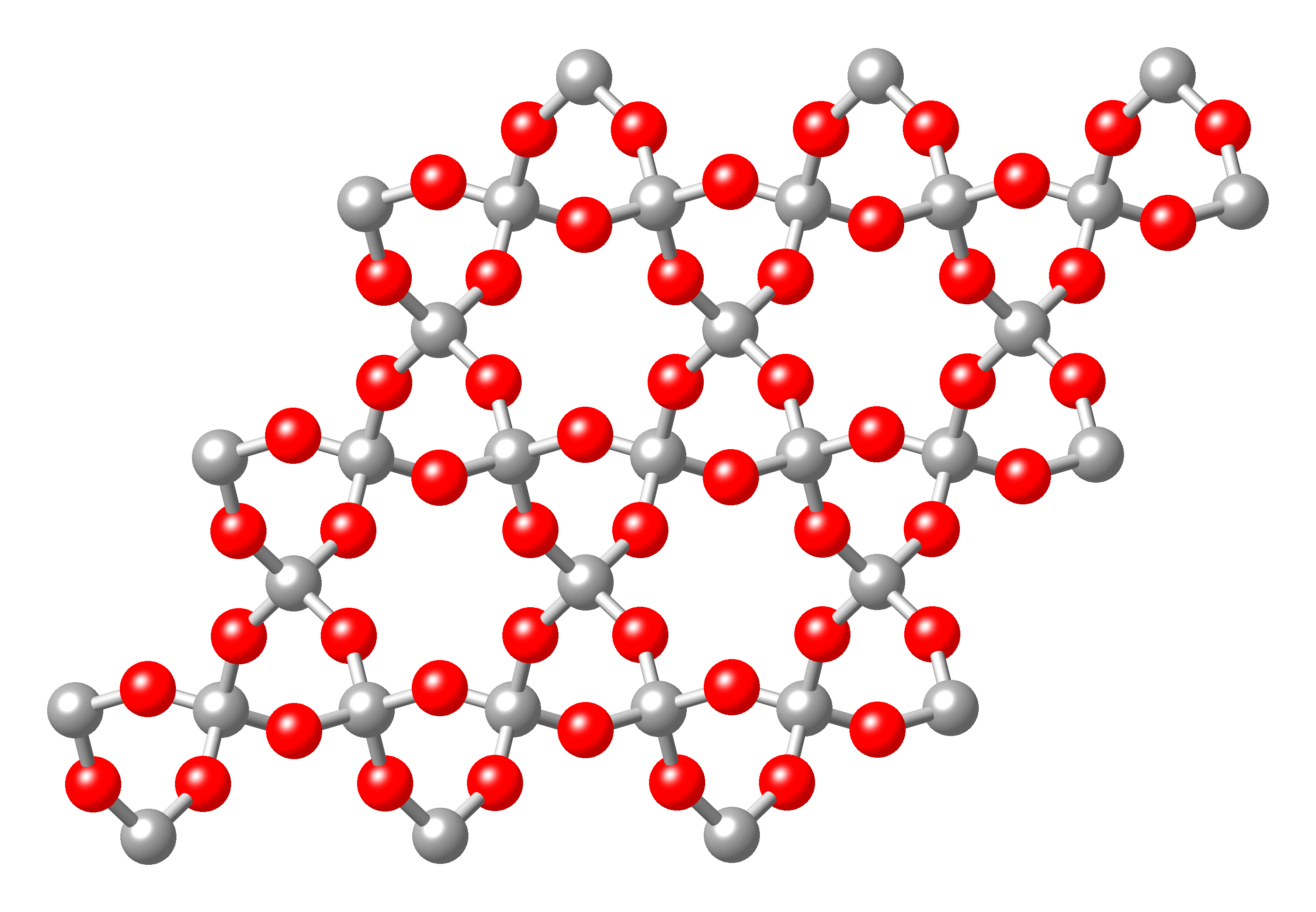
Ball-and-stick model of part of the crystal structure β-quartz, a form of silicon dioxide, SiO2, by Ben Mills via Wikimedia Commons
Beryl has a ring structure, shown here both as atoms and in a tetrahedron representation.
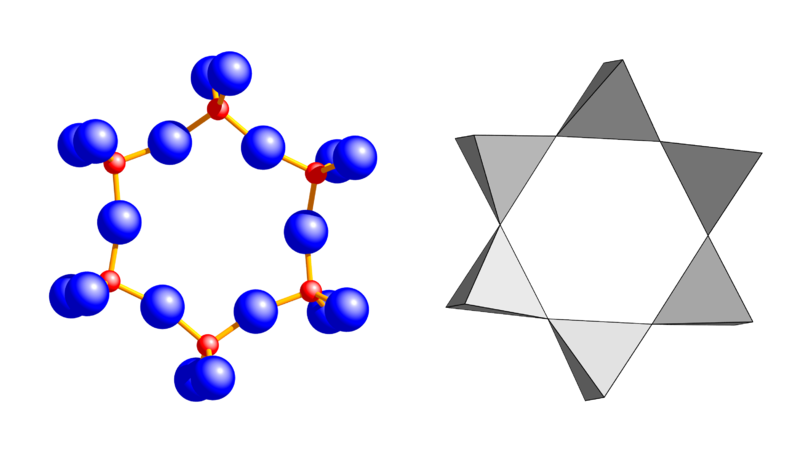
Unbranched 6er single ring of beryl from Brown and Mills via Wikimedia Commons
Non-silicate minerals (e.g., carbonates, sulfates and sulfides, oxides, etc.) make up only 5-8% of the Earth’s crust.
Rock cycle and types
As is well known, there are three types of rocks:
- igneous — formed when a molten rock precusor (magma or lava) cools;
- sedimentary — formed from mineral or organic particles, such as weathered or eroded rocks, which settle on the Earth’s surface or at the bottom of a body of water;
- metamorphic — formed by the transformation of preexisting rocks by intense heat or pressure, without melting.
Rocks may be intrusive (formed beneath the surface of the Earth) or extrusive (formed above the surface). Among igneous rocks, there are several types based on the amount of silica in them:
- felsic rocks contain > 65% silica and are generally light-colored;
- mafic rocks contain 45-55 % silica and are generally dark-colored;
- ultramafic rocks contain <45% silica.
Some common examples:
- granite — intrusive, igneous and felsic
- rhyolite — extrusive, igneous and and felsic
- basalt — extrusive, igneous and mafic
- sandstone — extrusive and sedimentary
- quartzite– intrusive and metamorphic
Rocks and minerals are formed in a series of cycles. Volcanic activity causes hot magma to pierce the Earth’s surface, where it cools and hardens into various sorts of igneous rocks. Other material may be coughed up from below ground at the same time as igneous or metamorphic rock. As these rocks are weathered by ice, water and wind, they are broken down into smaller grains which are then carried to the sea or lake floors, where they accumulate, eventually to form sedimentary rocks. These rocks may then be pushed by subduction down to a hotter, denser level where they are converted into igneous or metamorphic rocks and the cycle continues again2This is only one of the cycles of nature. It may be compared to, e.g., the water cycle (clouds – rain – bodies of water – evaporation – clouds) or the nitrogen cycle (fixation – assimilation – nitrification – denitrification).. Such cycles have been active for 4 billion years.
Interior structure of the Earth
The Earth consists of several layers. From the center:
1. The core, around 2500 km in radius, is composed of heavy metals such as iron and nickel and has two layers:
- the inner core, believed to be solid;
- the outer core, shown by seismic waves to be a very viscous liquid.
2. The layer outside the core is called the mantle and constitutes about 2/3 of the Earth’s mass. It is around 2800 km in radius and is composed mostly of light elements. Seismic studies show the boundary between core and mantle to be anything but smooth. The mantle has three layers:
- The mesosphere: Although temperatures here are high enough to melt the rock, the intense pressure keeps it solid.
- The asthenosphere: This layer is also solid, but can move, in a very slow plastic flow.
- The lithosphere: This is the outermost layer of the mantle and comprises both the outermost mantle layer and the crust. The lithosphere is rigid and relatively brittle. The mantle of the lithosphere is distinguished from the crust by its mineral composition.
3. Outside the so-called Moho discontinuity in the lithosphere lies the Earth’s crust, attached to the outer mantle. Above the Moho, the rocks are lighter and felsic, constituted primarily of silica, the principal constituent of surface rocks. There two kinds of crust:
- The thicker continental crust is about 20 km in depth (higher for mountains) and is composed mainly of granites, relatively light felsic rocks. The existence of dry land today depends on the existence of this relatively light rock.
- The thinner oceanic crust is typically less than 10 km thick, and is composed mainly of basalt, which is denser than continental crust.
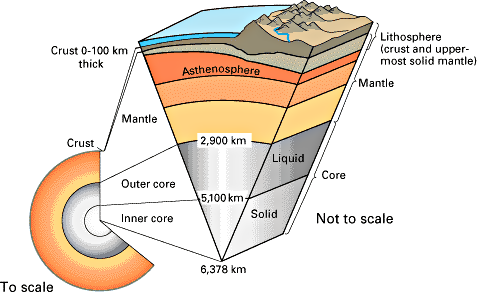
Earth’s core , from “This dynamic Earth” via USGS. Distances are depths, measured from the surface.
The relative densities of the two crust types are important in plate tectonics.
The Earth’s magnetic field
Because of its iron core, the Earth is like a bar magnet and has a dipole magnetic field with north and south poles. This is thought to be due to a dynamo effect, wherein charged particles in the hot core move about due to convection or the Earth’s rotation.
Actually, the polarity changes randomly in geologic time; the poles actually reverse. If this were to happen today, Antarctica would be found in the south! Fortunately for human navigation, this does not happen frequently, the last time having been about 780 thousand years ago. Geologists find a record of this reversing polarity in the phenomenon known as magnetic striping – variations in direction of the magnetic field of the rock on the ocean floor.
As it “burns”, the sun continually spews out charged particles, mostly electrons and protons, creating a solar wind. Fortunately for us, the magnetic field due to the Earth’s metal core deflects these particles so that most of them pass around the Earth without doing any harm. Otherwise, they could actually blow away the Earth’s atmosphere. Mars is much smaller than Earth and has lost its original molten core and magnetic field, so the solar wind has stripped away its atmosphere, leaving the red desert that space probes are exploring today.
As the solar wind passes over the Earth’s poles, where the magnetic field is most intense, they sometimes ionize the air and produce the beautiful wavy colors of the aurora.
The Earth’s magnetic field can deflect only a limited amount of the solar wind. In 1859, an enormous solar storm called the Carrington storm released so much electromagnetic energy and solar matter that aurora were seen as close to the Equator as the Caribbean and Santiago, Chile. Telegraph communication was often interrupted and operators reported sparks jumping from their equipment. If it happened again now, things like GPS satellites and telecommunications equipment could suffer.
Plate tectonics
Plate tectonics (from the Greek tecton, builder or carpenter) is the theory which explains how plates of continental crust move about on the surface of the Earth. It answers the question of how and why they move. It explains the existence of volcanoes and mountains and furnishes the background for all Earth science. It has been said to be the theory of “how the Earth works”. It has transformed and integrated modern geology just as quantum mechanics and relativity have modern physics or evolution, biology.
The idea of plates, continent-sized hunks of lithosphere moving on the surface of the earth, was first suggested by the comparison of the eastern border of South America with the western border of Africa. Since then, understanding of the Earth’s interior has explained how this works, although some details of the driving mechanism (especially, mantle plumes and hot spots) are still subjects of discussion .
The Earth’s lithosphere is composed of plates, seven main plates and numerous smaller ones. The plates are essentially huge hunks of rock centered around very ancient cores called cratons, most of which were formed during the Precambrian eons, perhaps as early as 4.3 Gya. They were certainly in existence by around 3.8 Gya, according to the age of sediments in western Greenland. Recent studies suggest that plate tectonics began about 3 Gya.3“New study zeros in on plate tectonics’ start date’, http://cmns.umd.edu/news-events/features/3404. The oceanic plates are thinner (less than 15km), but are composed mostly of very heavy basalt. The continental plates are thicker (up to 200km) but are composed mainly of granitic rocks, which are lighter. The plates essentially float on the asthenosphere, the next layer down, which is not a liquid, but can flow very slowly due to its high temperature.
It is currently thought that thermal convection due to the Earth’s hot core causes the plates to move very slowly about. This movement has caused the configuration of land – the continents – to change over geological history.
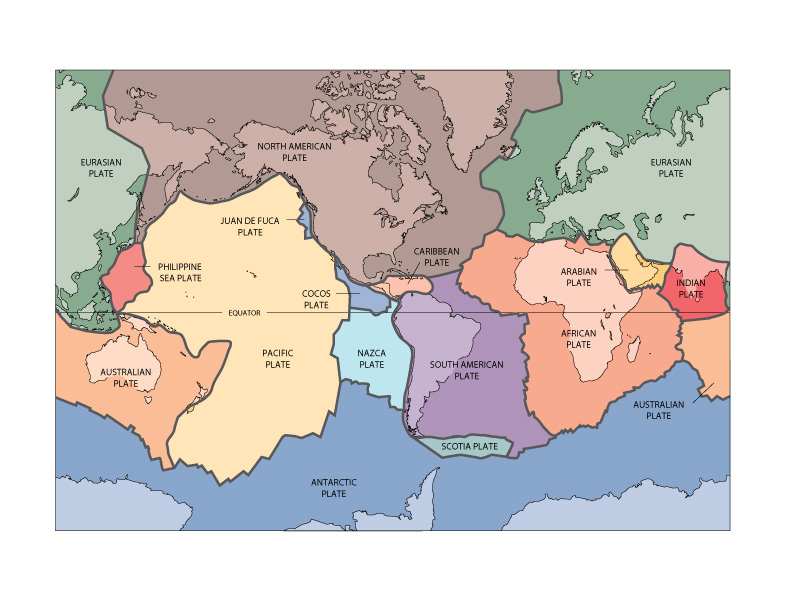
The Earth’s tectonic plates, from “This dynamic Earth” at USGS
When a plate of dense, oceanic rock collides edge-on with a plate of less dense continental rock, it is pushed down under the edge of the continental plate in a process called subduction. The descending ocean plate may create a deep trench at the boundary. Because of the heat from the rocks’ rubbing together and because water vapor released by the oceanic crust lowers the melting point of the rocks in contact with it, much of the rock melts, creating magma. Some of this rises through faults in the crust and a line of volcanoes may be formed along the edge of the continental plate above the subduction zone. The western coast of South America and much of that of North America are regions where the Pacific plate is being subducted under the American plates, forming the Andes and other mountains.
The Indian plate, which was once a separate continent south of Asia, moved northward and swung around to plow slowly into the southern edge of the Eurasian plate about 55 Mya. The impact between the two equally dense continental plates brought about no subduction, but instead pushed up the string of mountains known as the Himalayas. The process has not ended and the Himalayas are still growing.
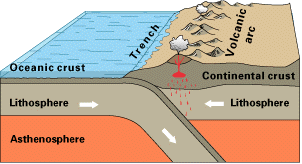
Subduction, from “This dynamic Earth” at USGS
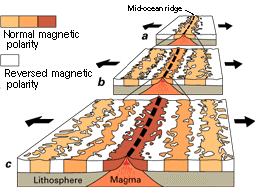
Ocean-floor magnetic striping, from “This dynamic Earth” at USGS
Volcanic processes deep in the Earth below the ocean beds can break through the thinner oceanic crust, letting magma escape. This magma usually forms basalt on cooling, so the Earth’s ocean floors are paved with dense basalt.
Right down the middle of the Atlantic Ocean, there is a ridge formed by magma pushed up by volcanic processes. As the magma flows out in east and west directions, perpendicularly to the ridge, the plates on either side grow at a rate of about 2.5 cm per year, so that their outer parts are pushed further east and west. In this way, the plates on either side of the Atlantic grow outwards from the mid-ocean ridge. In plate tectonics, this movement of two plates away from each other is called a divergent boundary or rift.4There is still some disagreement as to whether the rifting is pushing the plates apart or whether the moving plates are pulling away at the rift.
Not all magma moves to the side immediately. It tends to pile up, so that the central line of activity is higher than the surrounding area, forming an underwater mountain chain, such as the one down the center of the Atlantic. Running along the middle of the sub-oceanic chain, there is a trench where the magma comes out.
All the world’s oceans have come about in such a rifting process. In the case of the Atlantic Ocean, the oceanic and continental crusts on each side form single lithographic plates, so the above-water parts of the continental plates of the Americas and of Africa and Eurasia seem to move apart.5The mechanism explaining this phenomenon is still a subject of discussion. Since the surface area of the Earth remains the same, plates which are added to in one place must be destroyed elsewhere. As the Atlantic gets wider, so must the Pacific get smaller. This explains the widening or disappearance of oceans.
Magma also may break through the ocean floor at a single spot, know as a hot spot, bringing about the formation of a volcanic island above it. As the oceanic plate slides across the hot spot, a series of volcanoes may be formed, as is the case for the Hawaiian Islands or Icelandic volcanoes.
Molten magnetic rock solidifies in such a way that magnetic dipoles in it conserve the orientation of the Earth’s magnetic field. When the field reverses direction, so does the magnetization recorded in the rocks. The symmetric distribution about the trench of the magnetized rocks points clearly to spreading of the sea floor.
Plates may converge on each other, as in subduction along the Pacific shore of South America, or diverge from each other, as is probably happening in the Great African Rift, or slide alongside each other, as in the San Andreas Fault in California. Sometimes, two zones of continental crust may converge, piling up mighty mountain chains, as has been the case with the Pyrenees, the Alps or the Appalachians and is still going on in the Himalayas.
Iceland is a fascinating geological showplace where the mid-ocean mountain range has been pushed up above the water level by a local hot spot. Over time, the country has moved relative to the hot spot, leaving volcanic craters along the path. The whole island country sits astride the rift between the North American and European plates.
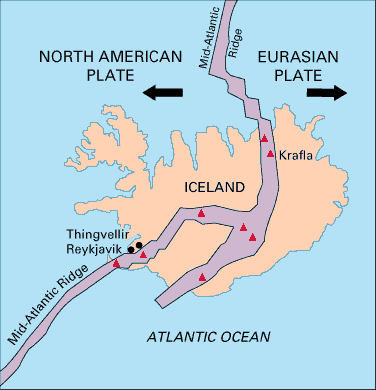
Iceland sits astride the joint of two plates, from “This dynamic Earth” at USGS
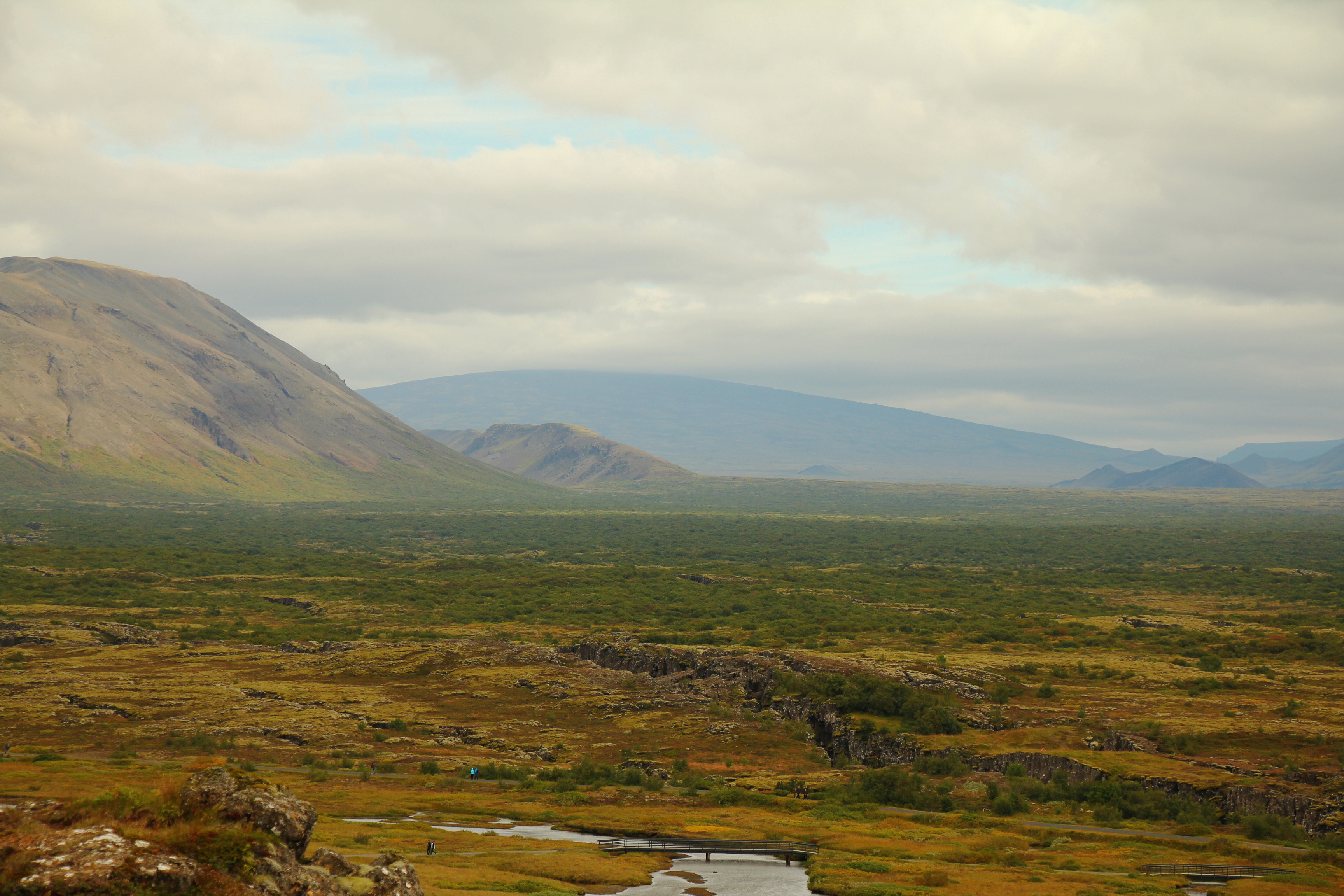
Looking out over the mid-Atlantic Ridge, the the rift between the American and European plates, at Þingvellir, Iceland. Photo by author.
Plate tectonics tells us that the continents we know have been in different configurations over the eons, sometimes separate, sometimes joined together into different large continents. At least once, they were joined together into a single supercontinent called Pangea, about which, more later.
In our solar system, plate tectonics is unique to Earth; no continental plates are known on other planets. Plate tectonics is of great importance for the evolution of living organisms.
The Earth’s atmosphere
Life on Earth depends on its atmosphere, a thin layer of gases, mostly nitrogen and oxygen, as well as some hydrogen and carbon and minute quantities of other elements. The atmosphere protects us and provides an environment suitable for life. It in turn depends on several other factors: the Earth’s temperature, its size (and, therefore, gravity) and its distance from the sun. In fact, in the beginning of Earth’s existence, the sun was not so bright, so temperatures were lower than one might expect..
What the atmosphere brings to the Earth
The atmosphere is important not only for its breathable gases but also for the protection it affords. It is essential in acting as a greenhouse gas to conserve the warmth from the sun. This effect can be overdone, as has been the case on Venus, which is much too hot to sustain life. The atmosphere also protects us from dangerous ultraviolet rays. And it shields us from countless meteorites, which are burned up from friction in the atmosphere before they can strike the ground and make huge craters, as has occurred on the atmosphere-less planet Mercury or on the Moon.
What the Earth brings to the atmosphere
The atmosphere is held onto the Earth by the gravitational force of the Earth itself. Earth’s mass and therefore its gravity is just right to hold onto the heavier gases nitrogen and oxygen that we breathe. In contrast, Mercury’s gravity is too weak to hold on to much of anything, so it has lost all its atmosphere. Jupiter, which is much larger than Earth, retains light gases as well, but packs them all down into concentrations such that its atmosphere is enormously dense. Tremendous storms rage across its surface, the most obvious one being the famous Red Spot.
The Earth’s size and its distance from the sun are delicately balanced. If the Earth were closer to the sun and therefore hotter, or if it were smaller and had less gravity, the movement of the atmosphere’s molecules would be great enough to allow them to escape the Earth’s gravitational attraction. Similarly, the relation between the production of heat by Earth’s core and the size of the Earth is crucial to maintenance of a temperature conducive to life. The temperature of the Earth’s surface also depends on its distance from the sun (closer would mean hotter) and its rotation (toasting each side evenly). The planet Venus is much closer to the Sun and its surface is burned up.
The Earth’s temperature and gravity guarantee conditions necessary to the existence of water in three phases – gas, liquid and solid – which are the source of all Earth’s weather and the rain cycles which irrigate the land. On Titan, one of Jupiter’s moons, analogous conditions hold for different reasons, namely that its atmospheric molecules are much cooler than Earth’s, so its lesser gravity is adequate to retain them. But the greatly reduced temperature relative to Earth’s means that the phases there are of methane, not oxygen. All the water on Titan is frozen hard as steel.
All these things — the hot magnetic core, the atmosphere, the distance from the sun, the size and the orientation of the Earth — work together to make life possible on our planet. Without any one of them, things would be a lot different. The Earth – and we, ourselves – must be considered privileged to be in a set of conditions propitious for life.
The carbon cycle
This seems as good a place as any to discuss the carbon cycle.1 It is a good deal more complex than the water cycle already presented.
Two major atmospheric components contain carbon: carbon dioxide (CO2) and methane (CH4), both greenhouse gases, but methane being far more powerful,
Geologists consider that carbon is exchanged among several types of carbon reservoirs:
- the atmosphere;
- the terrestrial biosphere;
- oceans;
- soil and sediments;
- Earth’s interior (mantle and crust).
The carbon cycle consists of a number of processes occurring in these reservoirs and exchanging carbon among them. Several such processes take place on land:
- Photosynthesis absorbs atmospheric CO2 and converts it into organic carbon in the biosphere (ejecting oxygen into atmosphere).
- Plant death releases carbon either to the atmosphere as CO2 or to the soil or sediment.
- Carbon in oxygen-poor soil may be transformed by bacteria and released to the atmosphere as methane, or it may be stored as sediments, peat or coal.
Processes of the carbon cycle in the sea include the following:
- Photosynthesizing plankton near the surface store carbon from the atmosphere as organic carbon;
- they also use dissolved carbon to make shells (CaCO3).
- When the plankton die, shells and organic carbon sink, removing carbon from the upper sea. Water in the surface layer is then relatively depleted in CO2 and so dissolves more from the air. Some deep-sea carbon dissolves, while some sinks as sediment. This step constitutes a “biological pump”, pumping carbon from the atmosphere, through the upper sea to the lower.
In the atmosphere, CO2 content is in a “delicate balance” which can be influenced by external phenomena. For instance, an increase in seawater temperature causes more CO2 to be released into the atmosphere; decrease,d temperature enhances absorption.
Over the long term, volcanic activity adds CO2 to the atmosphere and the resulting greenhouse effect increases atmospheric temperature. CO2 dissolves in rainwater to make carbonic acid (H2CO3). The acid and the increase in temperatrue both cause increased chemical weathering of rocks. The rock particles are then laid down as sediment which may later be subducted and eventually be ejected by volcanoes. The cycle then repeats.
So a long-term equilibrium between volcanic activity and chemical weathering (and other factors) maintains a balance of atmospheric CO2 over time.
O course, human emissions (fossil-fuel burning, livestock, etc) release CO2 and other greenhouse gases into atmosphere, leading to rises in global temperatures.
How do we know all this?
Geological clues come from different sources.
- Volcanic and other magma activity, along with measurement of seismic waves (which may be artificially induced) can show the configuration of the Earth’s mantle.
- Various rock formations, folds and magnetic properties give evidence of the action of plate tectonics. Evidence for plate tectonics also comes from fossils in the plates, especially along edges of plates which were formerly contiguous. The mid-oceanic trenches have been measured by sonar-equipped ships and by satellite.
- Quantities as diverse as the distribution of elements (e.g., iridium) and isotopes (e.g, oxygen) can give evidence about oxygen concentration or water temperatures.
- Measurement of remaining quantities of radioactive isotopes of certain elements with known decay rates can be used to determine the time which has passed since the element was incorporated into an object, rock or fossil. This method has been used in combination with fossil dating to determine the sequence of geological events described in this document.
- Fossils show the evolution of living beings and can be used to establish relative dates of the rocks in which they are found.
Now on to the first eon, the Hadean.
Notes
↑1 | Marshak, 142. |
---|---|
↑2 | This is only one of the cycles of nature. It may be compared to, e.g., the water cycle (clouds – rain – bodies of water – evaporation – clouds) or the nitrogen cycle (fixation – assimilation – nitrification – denitrification). |
↑3 | “New study zeros in on plate tectonics’ start date’, http://cmns.umd.edu/news-events/features/3404. |
↑4 | There is still some disagreement as to whether the rifting is pushing the plates apart or whether the moving plates are pulling away at the rift. |
↑5 | The mechanism explaining this phenomenon is still a subject of discussion. |